Thermal Energy, Temperature, and Heat
Thermal Energy, Temperature, and Heat
Knowledge of thermodynamic principles is essential for understanding chemistry, and indeed, all scientific disciplines. In fact when answering a question in chemistry that begins with the word “why”, the answer will likely require a thermodynamic explanation. The laws of thermodynamics are fundamental to natural science. Our lives are governed by these laws, but there is no penalty for breaking them, because as far as we know they cannot be broken.
The phrases thermal energy, temperature, and heat are often used interchangeably and incorrectly. To a chemist, these concepts have very different meanings. To illustrate the difference, we’ll examine the thermal energy, temperature, and heat associated with 1.0 g of water at 50 °C and 100.0 g of water at 50 °C.
Thermal Energy
Thermal energy is the kinetic energy associated with the random motion of atoms and molecules. This molecular motion can include translation (directional movement), rotation (tumbling), and vibration (stretching and flexing along the bond axis between two atoms in a molecule), shown in Figure 1. Thermal energy is an extensive property, meaning that the amount of the substance matters. Referring to our water example, the 1.0 g of water at 50 °C has 100 times less thermal energy than the 100.0 g of water at 50 °C because there are fewer molecules in motion in the smaller sample.
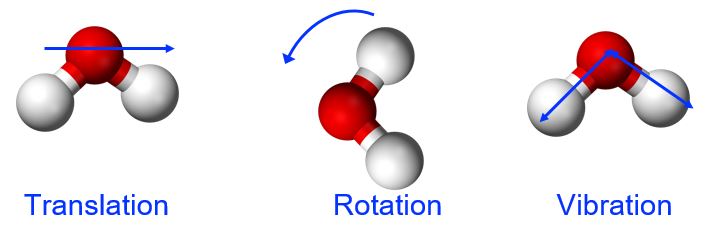
Temperature
Temperature is a quantitative measure of “hot” or “cold.” Recall from earlier that temperature is a measure of the average translational kinetic energy per molecule in a gas, liquid, or solid. Referring to our water example, the 1.0 g of water at 50 °C has the same temperature as the 100.0 g of water at 50 °C, even though the thermal kinetic energy is 100 times greater in the 100.0 sample of water. Temperature is an intensive property, meaning that the amount of the substances does not matter.
When the atoms and molecules in an object are moving or vibrating quickly, they have a higher average kinetic energy (KE), and we say that the object is “hot.” When the atoms and molecules are moving slowly, they have lower average KE, and we say that the object is “cold” (Figure 2). Assuming that no chemical reaction or physical change occurs, increasing the amount of thermal energy in a sample of matter will cause its temperature to increase because the average KE of the sample increases. And, assuming that no chemical reaction or physical change occurs, decreasing the amount of thermal energy in a sample of matter will cause its temperature to decrease. Temperature is thus a measure of the average translational KE of the particles in a sample.
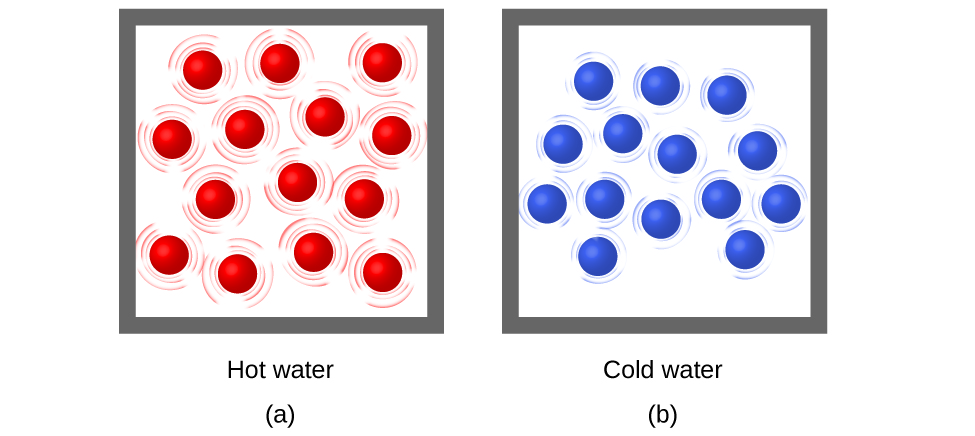
Heat
Heat (q) is the transfer of thermal energy between two substances at different temperatures. Referring to our water example, we cannot talk about the heat of the 1.0 g of water at 50 °C or the heat of the 100.0 g of water at 50 °C. Heat requires a flow of energy and results in a change in temperature; these examples are both stable at 50 °C. Even though the 100.0 g sample of water at 50 °C has a higher thermal energy than the 1.0 g sample of water at 50 °C, since there is not a temperature difference between the samples, no heat will flow.
Heat flow (a redundant term, but one commonly used) increases the thermal energy of one substance and decreases the thermal energy of the other (Figure 3). Suppose we initially have a high temperature (and high thermal energy) substance (H) and a low temperature (and low thermal energy) substance (L). The atoms and molecules in H have a higher average KE than those in L. If we place substance H in contact with substance L, the thermal energy will flow spontaneously from substance H to substance L. The temperature of substance H will decrease, as will the average KE of its molecules; the temperature of substance L will increase, along with the average KE of its molecules. Heat flow will continue until the two substances are at the same temperature (Figure 3).
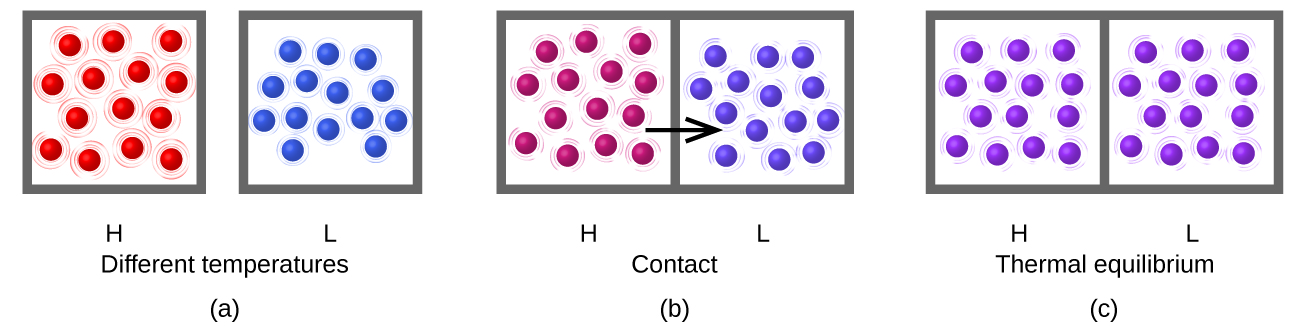
What is the mechanism of this energy transfer that we call heat? Energy is transferred through myriad random collisions of the hot molecules (red) with the walls of the container (gray box, e.g. iron), whose own atoms vibrate and collide with the molecules of the cold gas (blue). These collisions transfer energy from the hot molecules to the cold molecules because the hotter molecules move faster than colder ones (if they have the same mass), and thereby speed them up when they collide. The hotter molecules cool down (lower average kinetic energy per molecule and therefore lower temperature) and the colder molecules warm up (higher average kinetic energy per molecule and therefore higher temperature). No more energy is transferred (no heat flows) when the blue and red molecules have the same temperature and therefore the same average kinetic energy per molecule!
System vs. Surroundings
The measurement of heat transfer requires the definition of a system (the substance or substances undergoing the chemical or physical change) and its surroundings (everything else in contact with the system). Together, the system and surroundings constitute the universe from a thermodynamic perspective. Although there is heat transferred between the system and surroundings, there is no heat transferred into or out of the universe and quniverse is zero.
quniverse = 0 = qsystem + qsurroundings
We can rearrange this equation to state that:
qsystem = –qsurroundings
This relation just says that the energy that flows out of or into the system must exactly balance the energy that flow into or out of the surroundings. They must sum to zero. For example, if we measure the heat change in the water surrounding a chemical reaction, then we know the heat change in the chemical reaction is equal in magnitude and opposite in sign. Note that in this example the system can be the reactants and products dissolved in water, while the surroundings are the water itself.
In an endothermic reaction, energy flows into the system. Thus, heat is positive (q > 0) from the point of view of the system. Heat is transferred from the surroundings into the system. In contrast, in an exothermic reaction, energy flows out of the system. Thus, heat is negative (q < 0) from the point of view of the system. Heat is transferred from the system into the surroundings. Figure 4 illustrates heat flow.
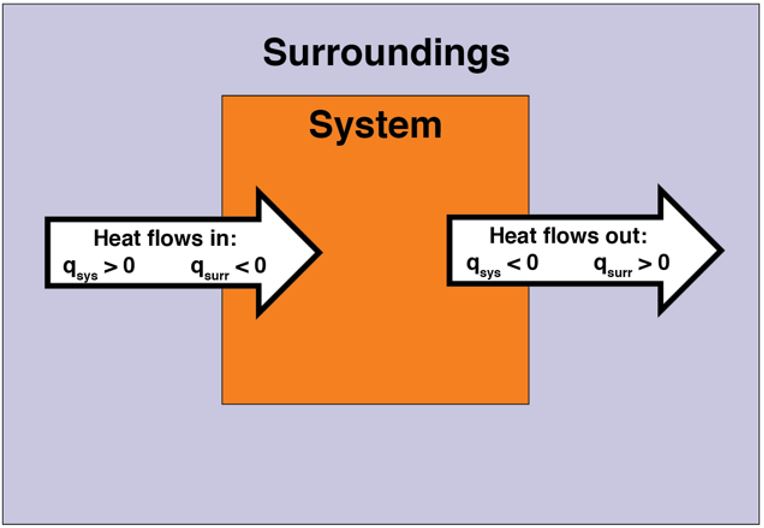
Example 1
When frost hits fruit growers at crucial periods, farmers spray mist on their crops to keep them from cooling to the air temperature. Determine the direction of heat flow when liquid water freezes to form solid water:
H2O(ℓ) ⟶ H2O(s)
Solution
As water freezes, intermolecular interactions are formed between water molecules and the physical change is exothermic; heat flows from the system to the surroundings. Here, the system is the water on the surface of the fruit and the surroundings includes the fruit. Heat flows from the water to the fruit, keeping the fruit from cooling to the air temperature which can be much lower than the ) 0 °C temperature at which pure water freezes.
Check Your Learning
Determine the direction of heat flow when hydrogen gas is ignited in the presence of oxygen gas to from water vapor.
2 H2(g) + O2(g) ⟶ 2 H2O(g)
Watch the demonstration here (start video playback around 1:10):
Answer
This is an exothermic chemical reaction; heat flows from the system to the surroundings. Here, the system includes everything inside the balloon and the surroundings includes everything outside the balloon. The flame is necessary to start the reaction, but it is ignored when discussing the heat flow.