D6.9 Proteins
Proteins are condensation polymers made from amino acids. An amino acid consists of a carbon atom (called the α carbon, shown in red below) bonded to a hydrogen atom, an amine group, a carboxylic acid group, and an R group, often called the side chain .
Notice that the α carbon is chiral when the “R” group differs from the other three groups. About 500 naturally occurring amino acids are known, although only twenty are found in protein molecules in humans. Of these twenty amino acids, nineteen have a chiral α carbon.
Exercise: Features of Amino-Acid Molecules
Amino acids are linked together in proteins by amide linkages formed via condensation reactions. An amide linkage in a protein is often called a peptide bond.
Note that the reaction does not involve the α carbon atom, and the chirality at the α carbon remains the same after the reaction. (In the product molecule above, R2 is depicted with a dashed bond because the amino acid shown in blue has been rotated 180° around a horizontal axis from the reactant to the product.)
Like all amide functional groups, the amide linkages in a protein strand are planar. The α carbon atoms, however, have tetrahedral local geometry and, at room temperature, there is free rotation around both single bonds to the α carbon atom. Thus, the protein chain is flexible and can fold into many different 3D shapes. This molecular chain of α carbon atoms connected by amide groups, is referred to as the protein backbone. It is the main chain of a strand of protein. The amino acid R groups are called side chains because they are attached to, but branch off from, the main chain.
Amino Acids
The twenty amino acids are often sorted into groups, for example, hydrophobic vs. hydrophilic, depending on the nature of their side chains. (The terms hydrophobic and hydrophilic refer to whether a side chain has intermolecular forces that are different from water or are similar to water, respectively.)
The nine amino acids that have hydrophobic side chains are shown in Figure: Amino acids with hydrophobic side chains.
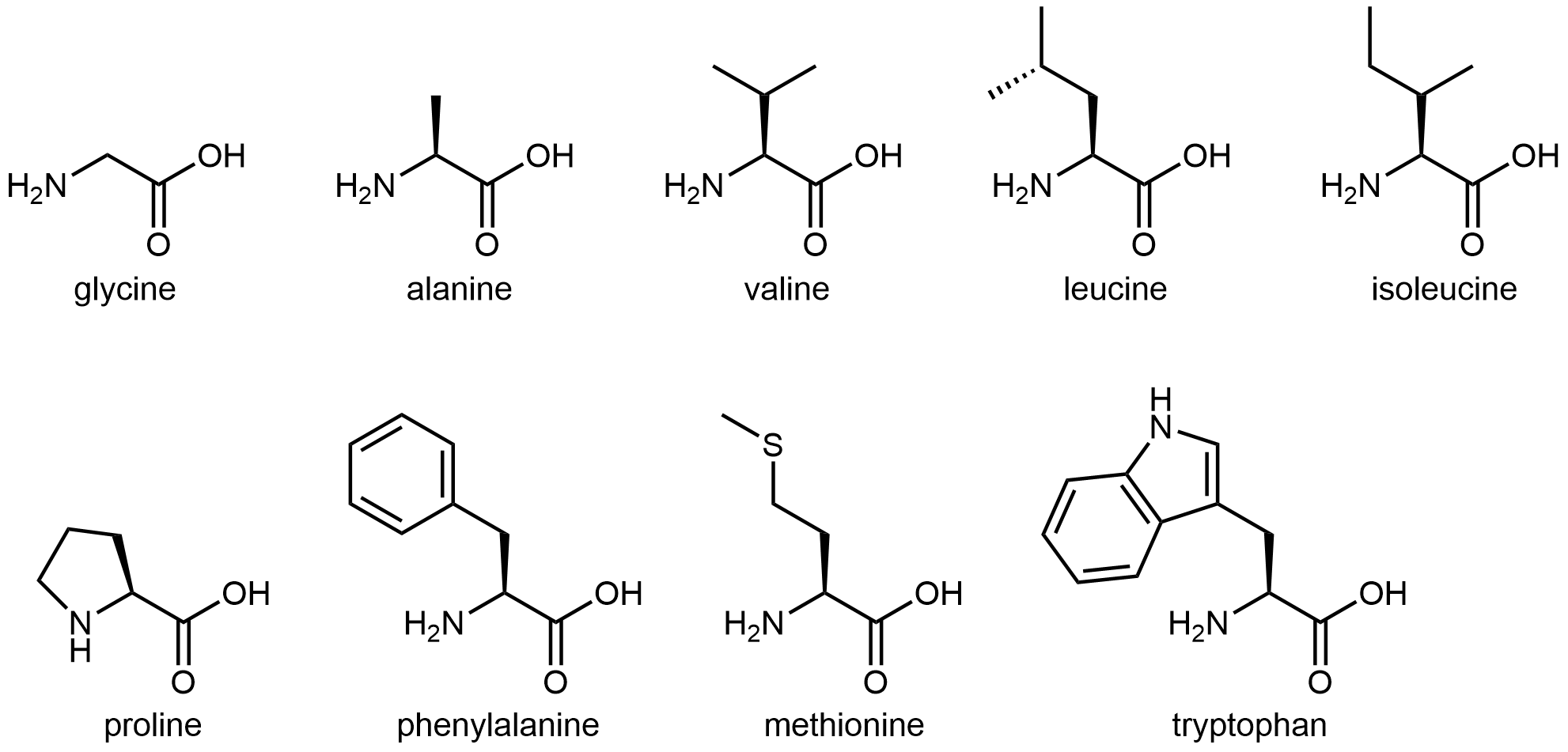
These hydrophobic side chains are composed mostly of carbon and hydrogen, have very small dipole moments, and tend not to mix with water molecules. As we will see, this characteristic has important implications for a protein’s structure.
The eleven amino acids shown in Figure: Amino acids with hydrophilic side chains have side chains that contains a functional group with significant dipole moment and often with strong hydrogen bonding capability. These intermolecular forces are similar to those between water molecules, so these side chains are hydrophilic.

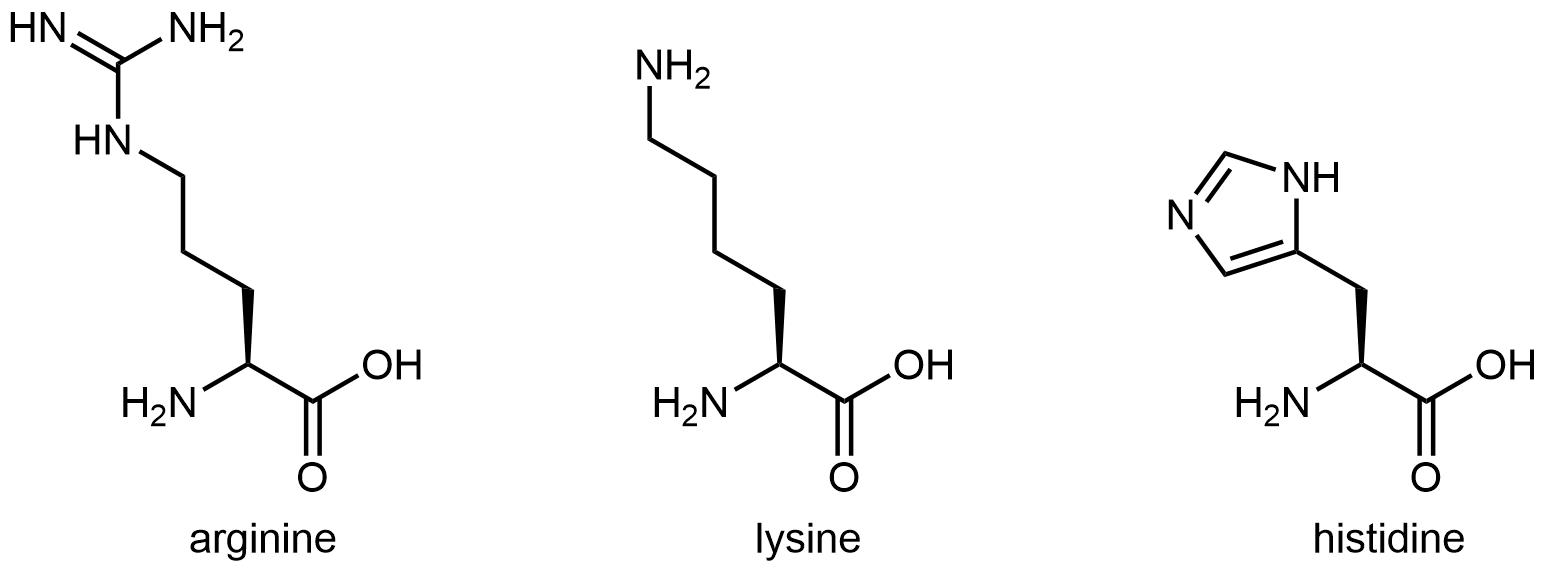
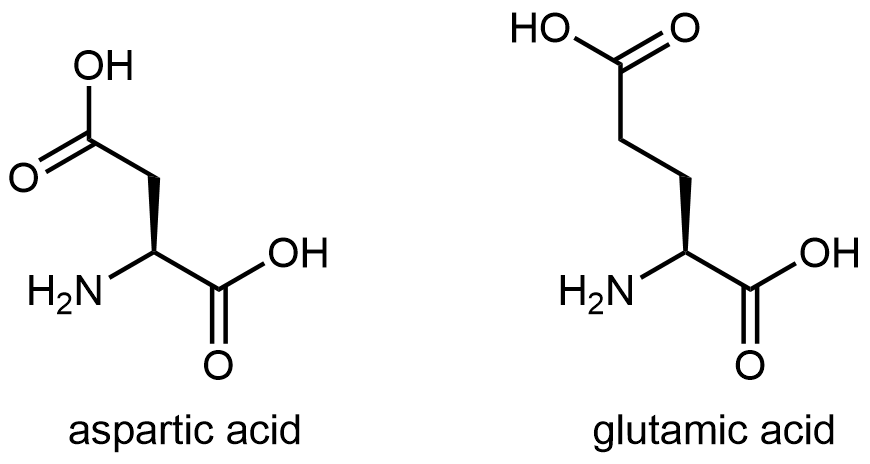
Exercise: Hydrophobic and Hydrophilic Side Chains
Protein Structure
The most important aspect of protein structure is the sequence in which the amino acids are linked: the primary structure. Because there are 20 possibilities for each side chain (highlighted in red below), for a given set of amino acids there many, many different protein primary structures possible. The unique three-dimensional secondary, tertiary, and quaternary structures described below all are determined by a protein’s primary structure.
By convention, protein sequences are written from the end with the free amine group (the N terminus) to the end with the free carboxyl group (the C terminus). For example, alanine-serine (Ala-Ser) differs from serine-alanine (Ser-Ala).
Segments of a long protein strand can adopt unique three-dimensional secondary structures. The type of secondary structure is defined by the pattern of hydrogen bonds between non-adjacent backbone amide groups. Two common elements of secondary structure are present in over 60% of known proteins. One is the α-helix, which has a regular pattern of hydrogen bonding between backbone amide groups.
For the segment shown above, the C=O of amino acid 1 forms a hydrogen bond with N-H of amino acid 5; the C=O of amino acid 2 forms a hydrogen bond with N-H of amino acid 6; etc. This regularly repeating hydrogen bonding is a prominent characteristic of an α-helix.
The other type of secondary structure is the β-sheet, which consists of stretches of the protein chain connected side-by-side by hydrogen bonds between backbone amide groups. This gives rise to a general pleated sheet appearance; you can see the pleated sheet by hiding the ribbon and rotating the figure below around a horizontal axis.
The tertiary structure of a protein is the overall three-dimensional shape of the protein. Figure: Tertiary structure shows one example of a globular tertiary structure, the protein myoglobin.
There are many ways that a protein polymer chain with a specific primary structure could be compressed and folded into a tertiary structure, but usually only a single structure exists. This lowest-energy structure is maintained by intermolecular forces involving different parts of the polymer chain., The primary forces that stabilize a protein’s three-dimensional structure are:
- Keeping hydrophobic side chains away from water as much as possible; for example, in the interior of a globular, water-soluble protein; orange side chains in the figure are mostly in the interior of the structure
- Maximizing London dispersion forces by minimizing open spaces in the interior of proteins
- Maximizing hydrogen bonding; for example, in α-helices or β-sheets
- Attractions between negatively and positively charged sites formed when acidic and basic side chains lose and gain H+ ions (discussed in more detail later in this course)
- Formation of disulfide bonds
Most of these should be familiar based on earlier descriptions of intermolecular attractive forces and ionic bonding. The last one, a disulfide bond, is similar to the cross-linking we discussed for addition polymers and involves covalent bonding between two sulfur atoms.
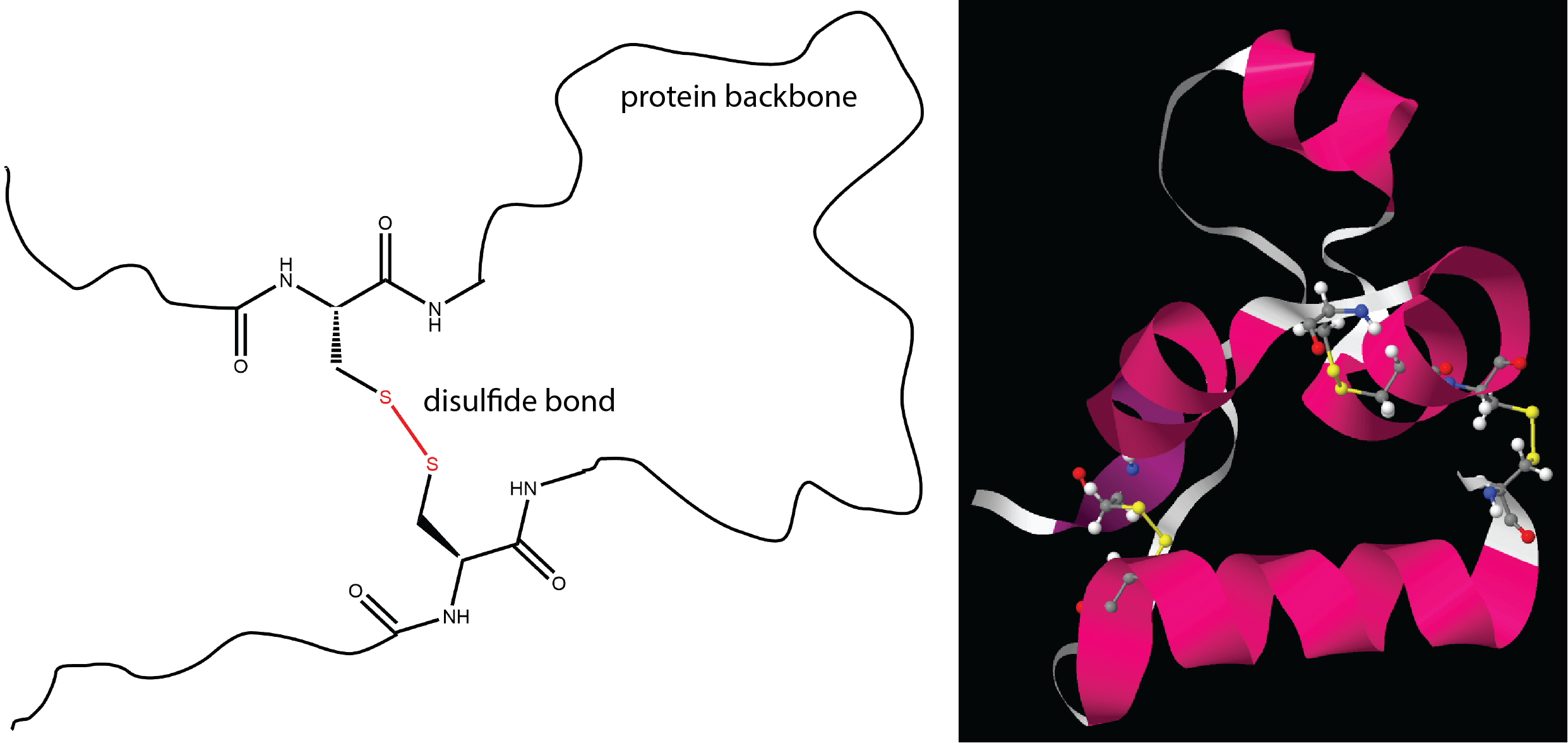
When two or more strands of proteins are held together by intermolecular forces, they can also adopt specific quaternary structures. For example, the hemoglobin in your blood is composed of four chains (colored peach, blue, green, and yellow in the figure below). The chains are held together by intermolecular forces, not by covalent bonds.
Please use this form to report any inconsistencies, errors, or other things you would like to change about this page. We appreciate your comments. 🙂 (Note that we cannot answer questions via the google form. If you have a question, please post it on Piazza.)