D5.4 Functional Groups
Aldehydes
An aldehyde or a ketone contains a carbonyl group, a carbon atom double bonded to an oxygen atom. The carbon atom in a carbonyl group is called the carbonyl carbon. In an aldehyde functional group, the carbonyl carbon is also bonded to a hydrogen atom. Hence, an aldehyde group can at most bond to one R group, and the aldehyde group is always at the end of a chain of carbon atoms.
Ketones
A ketone functional group consists solely of the carbonyl group. It bonds to two R groups, which may be the same or different, and is found partway along a chain of carbon atoms.
The reactivity of both aldehydes and ketones are directly related to the reactivity of the carbonyl group.
Formaldehyde, the simplest aldehyde, is a colorless gas with a pungent and irritating odor. It is sold in an aqueous solution called formalin, which contains about 37% formaldehyde by mass.
Formaldehyde causes coagulation of proteins, so it kills bacteria (and any other living organism) and stops many of the biological processes that cause tissues to decay. Thus, formaldehyde is used for preserving tissue specimens and embalming bodies. It can also be used to sterilize soil or other materials. Formaldehyde is used in the manufacture of Bakelite, a hard plastic having high chemical and electrical resistance.
Dimethyl ketone, CH3COCH3, commonly called acetone, is the simplest ketone. It is a colorless liquid that can be made commercially by fermenting corn or molasses. Among its many uses are: as a solvent for lacquer (including fingernail polish), cellulose acetate, cellulose nitrate, acetylene, plastics, and varnishes; as a paint and varnish remover; and as a solvent in the manufacture of pharmaceuticals and chemicals.
Exercise: Ketone Hybridization and Local Bond Geometry
Ethers
An ether functional group contains the group –O–, which bonds to two different R groups and is found in the middle of a molecule.
Diethyl ether, the most widely used ether, is a colorless, volatile liquid that is highly flammable. It was first used in 1846 as an anesthetic, but better anesthetics have now largely taken its place. Diethyl ether and other ethers are now used primarily as solvents for gums, fats, waxes, and resins. Methyl tert-butyl ether (abbreviated MTBE) is used as an additive for gasoline. MTBE belongs to a group of chemicals known as oxygenates due to their capacity to increase the oxygen content of gasoline.
Exercise: Ether Hybridization and Local Bond Geometry
Esters
An ester functional group contains a carbonyl group with a second oxygen atom single bonded to the carbonyl carbon and also single bonded to another carbon atom. A general ester structure has an R group bonded to the carbonyl carbon atom and another R group bonded to the second oxygen. It is a functional group that is found in the middle of a molecule.
The ester functional group’s carbon atom is sp2 hybridized with a trigonal planar local geometry. Its carbonyl oxygen is sp hybridized, and one of its unhybridized 2p atomic orbitals forms the π bond with the carbon’s unhybridized 2p atomic orbital. This oxygen also has two lone pairs: one occupies a sp hybrid orbital; the other occupies a 2p atomic orbital that is perpendicular to the π bond. The second oxygen (non-carbonyl oxygen) is sp2 hybridized and has a bent local geometry. It also has two lone pairs, one in a sp2 hybrid orbital, the other in the unhybridized 2p atomic orbital.
As shown in the preceding figure, the 2p lone pair on the non-carbonyl O is aligned parallel to the 2p orbitals that form the π bond. This leads to some delocalization of the lone pair electron densities, which can be expressed by resonance structures:
While the resonance structure on the right makes only a fairly minor contribution to the description of the ester molecule, that structure is helpful in understanding the ester’s chemical and physical properties. For example, the -COO- ester group is planar, and the non-carbonyl C-O bond is not as freely rotatable as a typical single bond. Moreover, an ester’s reactivity is quite different from that of a ketone or an ether, and hence an ester is a distinct functional group.
Activity: Ester Hybridization and Local Bond Geometry
The odors of ripe bananas and many other fruits are due to the presence of esters.
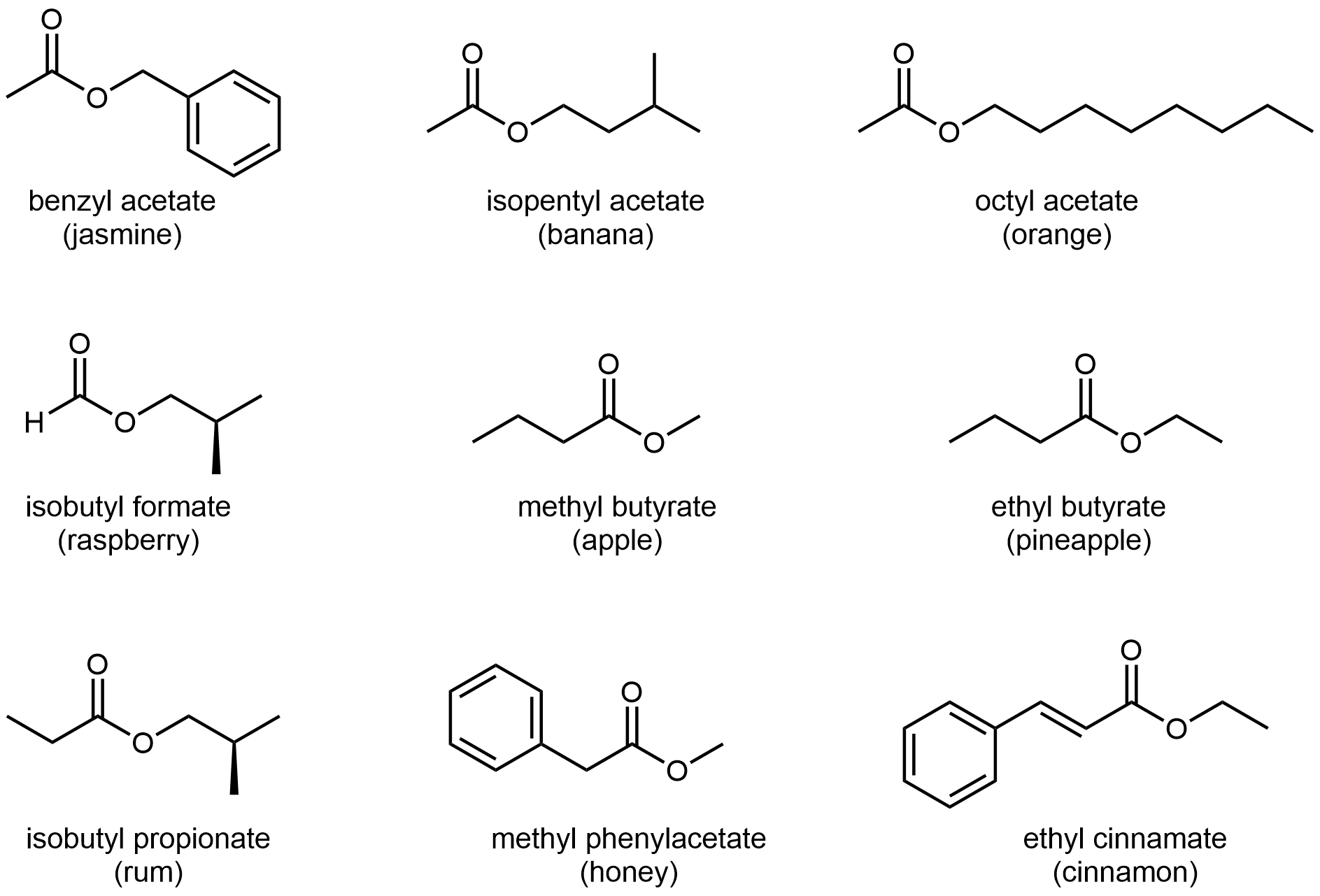
Alcohols
An alcohol functional group contains a hydroxyl (–OH) group covalently bonded to a carbon atom. (Note that the –OH group is covalently bonded; the OH is not a hydroxide ion.) The names of alcohols are derived from the names of the corresponding alkanes by removing the final “e” and adding “ol”. For example, the structure of the simplest alcohol is derived from the structure of methane by replacing one H atom with an OH group (CH3OH). It is named methanol.
Ethanol is produced by some species of yeast that catalyze fermentation of various sugars:
Ethanol can also be synthesized using the addition reaction of ethene and water using an acid as a catalyst:
This reaction is called an addition reaction because all atoms contained in the reactant molecules are contained in (added to) the product molecule. (In the reaction above, the two C atoms and four H atoms in ethene as well as both H atoms and the O atom in water are in the CH3CH2OH product molecule.)
Exercise: Structures of Alcohols
Knowing how to systematically name compounds can be helpful, especially when trying to decipher whether the line structures shown are constitutional isomers or not. See the nomenclature appendix for additional descriptions on how to name alcohol compounds. (You will not be explicitly tested on nomenclature in this course.)
Alcohol molecules can be classified according to the number of alkyl groups attached to the carbon with the -OH group, as shown in the figure below. If one alkyl group (or only hydrogen) is attached to that carbon, the alcohol is a primary (1º) alcohol. If two alkyl groups are attached, the alcohol is a secondary (2º) alcohol. If three alkyl groups are attached, the alcohol is a tertiary (3º) alcohol.
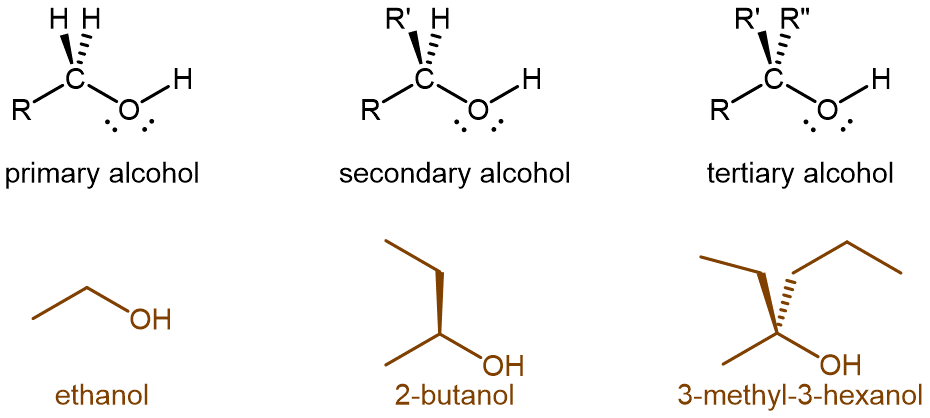
Exercise: Identifying Alcohols from Structure
Carboxylic Acids
A carboxylic acid functional group, -COOH, has a hydroxyl group linked to a carbonyl carbon atom. It differs from an ester in that the non-carbonyl oxygen is bonded to a hydrogen atom rather than an R group. Hence, carboxylic acid groups are found at one end of a molecule.
The simplest carboxylic acid is formic acid, known since 1670. Its name comes from the Latin word for ant, formicus. It is partially responsible for the pain and irritation of ants’ and wasps’ stings. Acetic acid is a main component (>4% by volume) of vinegar. Cider vinegar is produced by allowing apple juice to ferment without oxygen present; yeast changes sugar to ethanol, which is then converted to acetic acid via biological oxidation. Butyric acid, a component of rancid butter and Limburger cheese, has a vile odor.
As their name suggests, carboxylic acid groups are acidic. This is because their O-H bond can break relatively easily to yield -COO– and H+, allowing them to donate a proton, H+, to another molecule or ion. This acidity arises from the relative stability of the carboxylate anion, RCOO−.
Activity: Resonance Structures and Acidity of a Carboxylic Acid
Acetic acid (CH3COOH) is acidic because it can ionize to form the acetate anion and a hydrogen ion (proton):
CH3COOH ⟶ CH3COO− + H+
Draw the Lewis structure for acetate ion. Are there resonance structures for acetate anion? Remember that electron delocalization, as depicted in resonance structures, lowers the energy of a molecule or ion (stabilizes the molecue or ion).
Write a statement giving a reason that you agree with, or disagree with, this statement: Electron delocalization contributes to making a carboxylic acid more acidic than an alcohol (which also contains an –OH group).
Draw and write in your notebook, then left-click here for an explanation.
There are two important resonance structures for acetate ion.
The two structures have equal weight in the resonance hybrid. They show that the π bond is delocalized to both C-O bonds, and moreover the excess negative charge of the anion is stabilized by delocalizing to both (highly electronegative) oxygen atoms. As a result of this, when the carboxylic acid loses its proton, the carboxylate ion that forms has lower energy than if the excess negative charge is localized to a single atom. In an alcohol, when an anion forms, a single Lewis structure is sufficient to describe the anion where the excess negative charge is on the single O atom. There is no resonance stabilization and so an alcohol is less likely to lose its proton.
Molecules containing the carboxylic acid functional group are polar and can form hydrogen bonds. Pure acetic acid is called glacial acetic acid because its melting point of 16.6 °C is high enough that it can freeze in a cold laboratory. It is also quite thick and syrupy because the strong hydrogen-bonding attractions between molecules result in high viscosity. The acidity of the carboxylic acid group enhances the O-H···O hydrogen-bond strength, such that hydrogen-bonding between carboxylic acid molecules is usually stronger than between alcohol molecules. For example:
Amines
An amine functional group is a derivative of ammonia that contains one or more carbon-nitrogen bonds.
You can classify amine molecules by the number of C-N bonds they contain. In the above example, methylamine is a primary (1º) amine, dimethylamine is a secondary (2º) amine, and trimethylamine is a tertiary (3º) amine. In this figure methyl groups are shown but any R group, such as ethyl, could replace any of the methyl groups.
Like ammonia, amines are basic due to the lone pair on the nitrogen atom, and can undergo acid-base reactions to form protonated amine cations analogous to the ammonium ion NH4+:
For amines, 1º and 2º amines are capable of hydrogen bonding due to the presence of a N-H bond and the lone pair on the N atom, but 3º amines cannot form hydrogen bonds. For example, for the three amine isomers shown below, the boiling point of the 3º amine is significantly lower than the 1º and 2º isomers.
Amides
An amide functional group contains a nitrogen atom connected to the carbon atom of a carbonyl group. Like amines, amides can be classified by the number of carbon atoms bonded to the nitrogen:
Activity: Amines, Amides, and Resonance Structures
There is a crucial difference between amines and amides—there is an important resonance structure for the amide functional group that affects the molecular shapes, physical properties, and chemical properties of amides.
Draw a set of resonance structures for formamide (HCONH2). Based on the resonance structures you’ve drawn, determine the hybridization and local geometry of the N atom.
Draw and write in your notebook, then left-click here for an explanation.
The two resonance structures for formamide indicate that the nitrogen lone pair is involved in the π bonding network.
The delocalized π MO of formamide spans O, C, and N. Because the N lone pair is involved in the π bonding network, the lone pair must be in a p atomic orbital. Thus, the amide N atom is sp2 hybridized and has a trigonal planar local geometry. In fact, the whole formamide molecule is planar, as can be seen in this rotatable model.
Although the resonance structure with formal charges on O and N does not contribute to the resonance hybrid as much as the resonance structure without formal charges, it is crucial in understanding the chemical and physical properties of amide molecules. For example, the partial double bond character gives rise to a significant energy barrier for rotation about the C-N bond. And because the lone pair on the N atom is part of the π bonding network, the N atom in an amide is about 1010 times less basic than the N atom in an amine.
Additionally, because the lone pair on the N atom is part of the π bonding network, it is not available for hydrogen bonding. However, hydrogen bonds can still form in 1º and 2º amides between the N-H bond(s) and the lone pairs on the O atom.
Exercise: Structures of Amines
Exercise: Recognizing Functional Groups
Please use this form to report any inconsistencies, errors, or other things you would like to change about this page. We appreciate your comments. 🙂 (Note that we cannot answer questions via the google form. If you have a question, please post it on Piazza.)