49 Voltaic Cells (M18Q3)
Learning Objectives
- Identify the half-reactions occurring at the anode and the cathode in a voltaic cell, as well as the polarity of the electrodes, the direction of electron flow in the external circuit, and the direction of ion flow in the salt bridge.
- Use and interpret line notation for a voltaic cell.
| Line Notation |
| Key Concepts and Summary | Glossary | End of Section Exercises |
Consider what happens when a clean piece of copper metal is placed in a solution of silver nitrate (Figure 1). As soon as the copper metal is added, silver metal begins to form and copper ions pass into the solution. The blue color of the solution on the far right indicates the presence of copper ions. Electrons spontaneously move from the copper metal to the silver ions.
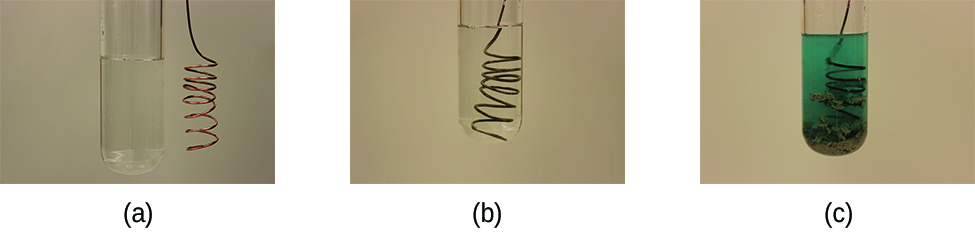
Galvanic or voltaic cells involve spontaneous oxidation reduction reactions in which the half-reactions are separated so that electrons, or current, can flow through an external wire (Figure 2).
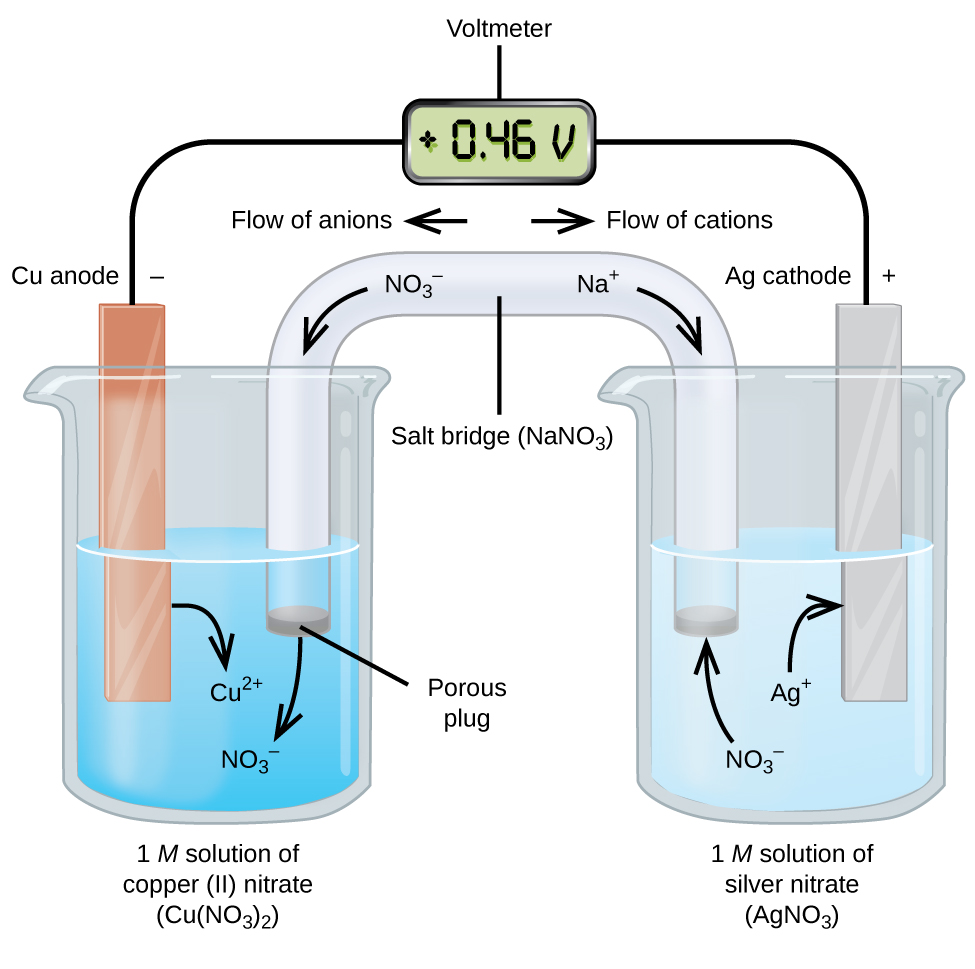
The beaker on the left side of the figure is called a half-cell, and contains a 1 M solution of copper(II) nitrate [Cu(NO3)2] with a piece of copper metal partially submerged in the solution. The copper metal is an electrode, a solid electrical conductor that carries electric current into non-metallic substances, such as aqueous solutions, gases, or non-metallic liquids and solids. The copper is undergoing oxidation; therefore, the copper electrode is the anode. The anode is connected to a voltmeter with a wire and the other terminal of the voltmeter is connected to a silver electrode by a wire.
The half-cell on the right side of the figure consists of the silver electrode in a 1 M solution of silver nitrate (AgNO3). The silver is undergoing reduction; therefore, the silver electrode is the cathode.
At this point, no current flows—that is, no significant movement of electrons through the wire occurs because the circuit is open. The circuit is closed using a salt bridge, which transmits the current with moving ions. The salt bridge consists of a concentrated, nonreactive, electrolyte solution, such as the sodium nitrate (NaNO3) solution used in this example. As electrons flow from left to right through the electrode and wire, nitrate ions (anions) pass through the porous plug on the left into the copper(II) nitrate solution. This keeps the beaker on the left electrically neutral by neutralizing the charge on the copper(II) ions that are produced in the solution as the copper metal is oxidized. At the same time, the nitrate ions are moving to the left, sodium ions (cations) move to the right, through the porous plug, and into the silver nitrate solution on the right. These added cations “replace” the silver ions that are removed from the solution as they were reduced to silver metal, keeping the beaker on the right electrically neutral. Without the salt bridge, the compartments would not remain electrically neutral and no significant current would flow.
The instant the circuit is completed, the voltmeter reads +0.46 V, this is called the cell potential. The cell potential is created when the two dissimilar metals are connected, and is a measure of the energy per unit charge available from the oxidation-reduction reaction.
When the electrochemical cell is constructed in this fashion, a positive cell potential indicates a spontaneous reaction and that the electrons are flowing from the anode to the cathode.
To summarize, in Figure 2:
- Electrons flow from the anode to the cathode: left to right in Figure 2.
- The electrode in the left half-cell is the anode because oxidation occurs here. The anions from the salt bridge flow towards the anode.
- The electrode in the right half-cell is the cathode because reduction occurs here. The cations from the salt bridge flow towards the cathode.
- In Figure 2, the cell potential of +0.46 V results from the inherent differences in the nature of the materials used to make the two half-cells.
- The salt bridge must be present to complete the circuit and both an oxidation and reduction must occur for current to flow.
There are many possible reactions used in galvanic cells, so a shorthand notation can be used to describe them. The line notation (sometimes called a cell notation) provides information about the various species involved in the reaction. This notation also works for other types of cells. A vertical line, │, denotes a phase boundary and a double line, ‖, the salt bridge. Information about the anode is written to the left, followed by the anode solution, then the salt bridge (when present), then the cathode solution, and, finally, information about the cathode to the right. The line notation for the galvanic cell in Figure 2 is then:
Note that spectator ions are not included and that the simplest form of each half-reaction was used. When known, the initial concentrations of the various ions are usually included.
Some oxidation-reduction reactions involve species that are poor conductors of electricity, and so an inert electrode is used that does not participate in the reactions. Frequently, the electrode is platinum, gold, or graphite, all of which are inert to many chemical reactions. One such system is shown in Figure 3. Magnesium undergoes oxidation at the anode on the left in the figure and hydrogen ions undergo reduction at the cathode on the right. The reaction may be summarized as
Oxidation, in anode cell: | Mg(s) → Mg2+(aq) + 2 e– |
Reduction, in cathode cell: | 2 H+(aq) + 2 e– → H2(g) |
Overall Reaction: | Mg(s) + 2 H+(aq) → Mg2+(aq) + H2(g) |
The cell used an inert platinum wire for the cathode. The platinum does not participate in the chemistry and is not included in the chemical equation, however, it is included in the line notation as follows:
Mg(s) | Mg2+(aq) || H+(aq) | H2(g) | Pt(s)
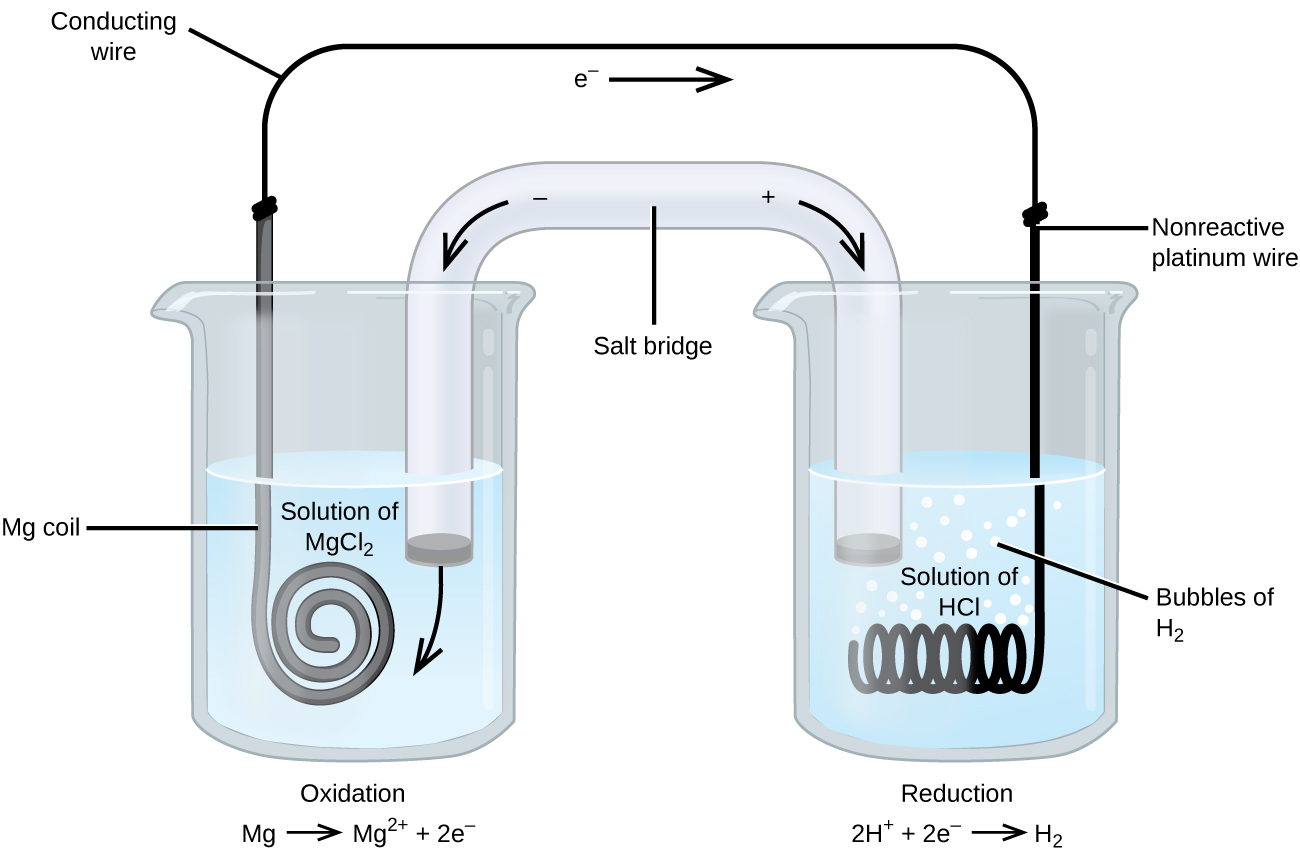
Example 1: Using Line Notation
1. Consider a galvanic cell consisting of
2 Cr(s) + 3 Cu2+(aq) → 2 Cr3+(aq) + 3 Cu(s)
Write the oxidation and reduction half-reactions and write the reaction using line notation. Which reaction occurs at the anode? The cathode?
Solution
By inspection, Cr is oxidized when three electrons are lost to form Cr3+, and Cu2+ is reduced as it gains two electrons to form Cu. Balancing the charge gives:
Oxidation, in anode cell: | 2 Cr(s) → 2 Cr3+(aq) + 6 e– |
Reduction, in cathode cell: | 3 Cu2+(aq) + 6 e– → 3 Cu(s) |
Overall Reaction: | 2 Cr(s) + 3 Cu2+(aq) → 2 Cr3+(aq) + 3 Cu(s) |
Line notation uses the simplest form of each of the equations (without coefficients), and starts with the reaction at the anode. No concentrations were specified so:
Cr(s) | Cr3+(aq) || Cu2+(aq) | Cu(s)
2. Consider a galvanic cell consisting of:
5 Fe2+(aq) + MnO4–(aq) + 8 H+(aq) → 5 Fe3+(aq) + Mn2+(aq) + 4 H2O(l)
Write the oxidation and reduction half-reactions and write the reaction using line notation. Which reaction occurs at the anode? The cathode?
Solution
By inspection, Fe2+ undergoes oxidation when one electron is lost to form Fe3+, and MnO4− is reduced as it gains five electrons to form Mn2+. Balancing the charge gives:
Oxidation, in anode cell: | 5 Fe2+(aq) → 5 Fe3+(aq) + 5 e– |
Reduction, in cathode cell: | MnO4–(aq) + 8 H+(aq) + 5 e– → Mn2+(aq) + 4 H2O(l) |
Overall Reaction: | 5 Fe2+(aq) + MnO4–(aq) + 8 H+(aq) → 5 Fe3+(aq) + Mn2+(aq) + 4 H2O(l) |
Line notation uses the simplest form of each of the equations, and starts with the reaction at the anode. It is necessary to use an inert electrode, such as platinum, because there is no metal present to conduct the electrons from the anode to the cathode.
Pt(s) | Fe2+(aq), Fe3+(aq) || MnO4–(aq), H+(aq), Mn2+(aq) | Pt(s)
Oxidation occurs at the anode and reduction at the cathode.
Check Your Learning
Use line notation to describe the galvanic cell where copper(II) ions are reduced to copper metal and zinc metal is oxidized to zinc ions.
Answer:
From the information given in the problem:
Oxidation, in anode cell: | Zn(s) → Cr2+(aq) + 2 e– |
Reduction, in cathode cell: | Cu2+(aq) + 2 e– → Cu(s) |
Overall Reaction: | Zn(s) + Cu2+(aq) → Zn2+(aq) + Cu(s) |
Using line notation:
Zn(s) | Zn2+(aq) || Cu2+(aq) | Cu(s)
Key Concepts and Summary
A common type of electrochemical cell is a galvanic or voltaic cell, which involves spontaneous redox reaction with electrons flowing through an external wire. Each half-cell has metal electrodes that carry the electric current from the anode, where oxidation occurs, to the cathode, where reduction occurs. In order to complete the circuit, a salt bridge is used to connect the aqueous phases of the anode and cathode and a flow of ions, anions toward the anode and cations toward the cathode, maintain charge neutrality. Line notation is often used as a shorthand way to describe a voltaic cell, rather than having to draw a full diagram.
Glossary
anode
the electrode where oxidation, or loss of electrons, occurs
cathode
the electrode where reduction, or gain of electrons, occurs
cell potential
a measure of the energy per unit charge available from the redox reaction
electrode
solid electrical conductor that carries electric current into non-metallic substances, such as aqueous solutions, gases, or non-metallic liquids and solids
galvanic or voltaic cells
electrochemical cell that involves a spontaneous redox reaction in which the half-reactions are separated so that electrons (or current) can flow through an external wire
line notation
a shorthand notation that provides information about the half-cells and which species is oxidized or reduced in an electrochemical cell
salt bridge
a concentrated, non-reactive electrolyte solution used to close the electrochemical circuit in a voltaic cell by transmitting current with the flow of ions
Chemistry End of Section Exercises
- Why is a salt bridge necessary in a voltaic cell?
- An active (metal) electrode was found to lose mass as the oxidation-reduction reaction was allowed to proceed. Was the electrode part of the anode or cathode? Explain.
- A voltaic cell has been assembled to use the following overall chemical reaction:
Fe(s) + Cu(NO3)2(aq) → Fe(NO3)2(aq) + Cu(s)
- Write the half reactions for each cell and indicate which reaction is occurring at the anode and which is occurring at the cathode.
- Fe(NO3)2 solutions are pale green and Cu(NO3)2 solutions are blue. What visual observations do you predict you will see as the voltaic cell reaction progresses?
- The two half-cells are connected by a salt bridge containing concentrated KNO3. Into which cell will each ion flow? Explain.
- Write the cell notation for this voltaic cell.
- A voltaic cell has been assembled to use the following overall chemical reaction:
Ni(s) + 2 Ag+(aq) → Ni2+(aq) + 2 Ag(s)
- Write the half reactions for each cell and indicate which reaction is occurring at the anode and which is occurring at the cathode.
- Determine the electron flow over an external wire
- The two half-cells are connected by a salt bridge containing concentrated KNO3. Into which cell will each ion flow? Explain.
- Write the cell notation for this voltaic cell.
Answers to Chemistry End of Section Exercises
- Without the salt bridge, the circuit would be open (or broken) and no current could flow. With a salt bridge, each half-cell remains electrically neutral and current can flow through the circuit.
- Oxidation reactions convert metal to metal ions, which pass into solution, so mass loss will be seen at the anode in a voltaic cell.
-
- Anode: Fe(s) → Fe2+(aq) + 2 e– ; Cathode: Cu2+(aq) + 2 e– → Cu(s)
Note that the NO3– ions are left out of the half-reactions because they are spectator ions in this process. - The anode half-cell will contain a pale green solution that will become more green as the reaction progresses and more Fe2+ ions are produced. The cathode reaction will contain a blue solution that will become more colorless as the reaction progresses and Cu2+ ions are consumed.
- As the reaction progresses, positive Fe ions are being produced so anions, NO3–, will flow from the salt bridge into the anode cell to keep the solution neutrally charged. At the same time, positive Cu ions are being consumed, so cations will flow from the salt bridge into the cathode cell to keep the solution neutrally charged.
- Fe(s) | Fe2+(aq) || Cu2+(aq) | Cu(s)
- Anode: Fe(s) → Fe2+(aq) + 2 e– ; Cathode: Cu2+(aq) + 2 e– → Cu(s)
- Oxidation reactions convert metal to metal ions, which pass into solution, so mass loss will be seen at te anode in a voltaic cell.
- Anode: Ni(s) → Ni2+(aq) + 2 e– ; Cathode: Ag+(aq) + e– → Ag(s)
- Electrons will flow from the oxidation half-cell, containing the Ni/Ni2+, to the reduction cell, containing the Ag/Ag+.
- As the reaction progresses, Ni cations are being produced so anions, NO3–, will flow from the salt bridge into the anode cell to keep the solution neutrally charged. At the same time, Ag cations are being consumed, so cations will flow from the salt bridge into the cathode cell to keep the solution neutrally charged.
- Ni(s) | Ni2+(aq) || Ag+(aq) | Ag(s)
Feedback/Errata