D19.1 Chemical Reactions
In Units 1 and 2 we discussed chemical substances and how their atomic-scale structure affects their properties. We also discussed physical processes, such as phase change or dissolving, where atomic-scale structure of the substance remains the same before and after. We now turn to chemical reactions processes that change one (or more) substance into one (or more) new substance. For example, grinding silver-gray zinc (Zn) metal into a powder is a physical change. If, at room temperature, this powdered zinc is mixed with powdered sulfur (S, which has a bright yellow color), the result is simply a mixture of zinc and sulfur. No chemical reaction occurs. However, raising the mixture’s temperature with a red-hot wire enables a chemical reaction: the zinc reacts with the sulfur to form zinc sulfide (ZnS).
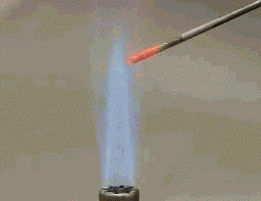
The reaction,
once started, transfers energy to its surroundings, as evidenced by the flash of light and hot sparks.
Such chemical changes involve redistribution of valence electrons. In the solid zinc metal, Zn valence electrons hold the atoms together via metallic bonding. In the sulfur solid, which exists as S8 molecules, valence electrons are involved in forming S-S covalent bonds. As zinc reacts with sulfur, these valence electrons rearrange: some electrons move from being near zinc atoms to being closer to sulfur atoms. The resulting ZnS is an ionic compound, where sulfur atoms have excess electron densities and zinc atoms are electron deficient.
Here is another example, the reaction of ammonia with strong acid in aqueous solution. This involves rearrangement of covalent bonds and lone pairs: the lone pair on N (red) forms a new N-H σ bond (green), and the O-H σ bond (red) breaks leaving an additional lone pair on O (green).

NH3(aq) + H3O+(aq) → NH4+(aq) + H2O(ℓ)
Notice that in both examples above, reactants combine in fixed ratios to achieve products. In a chemical reaction, atoms are neither created nor destroyed. An atom may be transferred from one molecule to another or it may gain or lose electrons to become an ion, but it cannot disappear. New atoms cannot form out of nowhere. This is the reason we balance chemical reactions.
Chemical changes are useful because they can change one kind of substance into another that is more useful for humankind. Thus it is important to know whether a given set of reactants can react to form products, how long it takes to form products, and how much product we can get. The remainder of this course is designed to enable you to predict whether reactions occur, to understand how reactions can be sped up or slowed down, and to determine how much product can be expected from a reaction.
One way to begin making such predictions is to classify a reaction as one of two models that describe how electron density is redistributed:
- Oxidation-reduction reactions involve the movement of electrons. For example, in the Zn(s) + S(s) → ZnS(s) reaction, electrons are transferred from Zn to S.
- Acid-base reactions involve the redistribution of lone pairs of electrons and breaking/forming of covalent bonds, as in the ammonia plus strong acid reaction above.
We will discuss these two types of chemical reactions in detail later in the semester. But first, we will consider reactions that reach equilibrium. Some questions we will address along the way are: why do some reactions produce lots of product while others produce little? Why can zinc powder and sulfur powder be mixed with no reaction at room temperature? (We can see from the figure above that this reaction occurs readily at a higher temperature and the transfer of energy to the surroundings indicates that the products are lower in energy than the reactants.)
As you will see, whether a reaction proceeds and the quantities of products produced both depend on energy—but not simply on whether products are lower in energy than reactants. We will develop the idea of Gibbs free energy, a property that includes not only energy but also how probable the distribution of energy is. Gibbs free energy can tell us whether reaction products will form and how much we will get.
Please use this form to report any inconsistencies, errors, or other things you would like to change about this page. We appreciate your comments. 🙂 (Note that we cannot answer questions via the google form. If you have a question, please post it on Piazza.)