Volcanoes
There are a number of distinctly different types of volcanoes. Before reading this section, go through the brief description of volcanoes, how they erupt, and what the main types are, at this US Geological Survey web page. Here we will discuss how these different types of volcanoes and the material they erupt can be related to topics discussed in class, on igneous rocks and plate tectonics.
Importance of the Silica Content of Magma
We’ll start with the relationships shown in Figure 1 below, used in discussing igneous rocks. Recall that it shows how silica content in magma is related to minerals in igneous rock and its density and other properties.
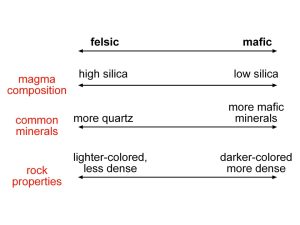
There are other important effects of magma silica content that have major impacts on volcanic eruptions. Gases are dissolved in magma but can separate from it to form bubbles as the magma moves toward the surface where it is under less pressure and cooler. When there are more gas bubbles and they can’t easily escape from the magma, the resulting eruption is much more explosive.
Explosive eruptions are more common with high-silica magma than with low-silica magma, for several reasons. High-silica magma has higher gas content and is also more viscous, that is, it does not flow as easily. Gas bubbles cannot easily escape through the viscous magma, so they build up and make conditions more explosive. The viscous nature of high-silica magma is partly because it’s cooler, but also because the silica itself increases viscosity. With that background, we can create another diagram, relating silica content to magma viscosity and explosiveness of the eruption (Figure 2).
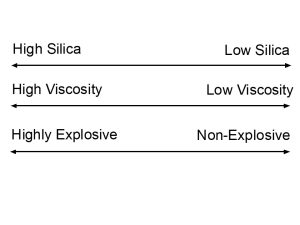
Materials Produced by Explosive and Non-Explosive Volcanic Eruptions
The following photos illustrate the difference between explosive and non-explosive eruptions, as well as common products of volcanic eruptions. In Hawaii, volcanic eruptions are produced by low-silica, mafic magma, and are relatively non-explosive. The major material produced by these eruptions is lava, molten rock that flows out of openings called vents and continues flowing downhill over the land surface before coming to a halt as it cools. Lava flows nowhere near as fast as water, but can eventually cover large areas if it keeps flowing. Lava is produced by magma ranging from low to high silica, but it large accumulations of lava are generally produced by non-explosive eruptions of low-silica magma, cooling to form mafic rocks like basalt.
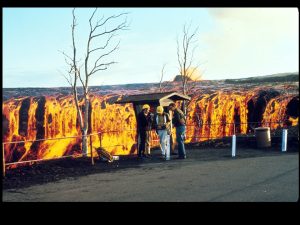
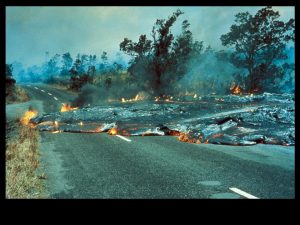
At times in the past, huge volumes of low-silica lava have erupted to cover large areas of land, cooling to form what are called flood basalts. Flood basalts covered a large part of what is now Oregon and Washington, mostly between 17 and 14 million years ago (Figure 5), although some eruptions continued until 5 million years ago. Instead of volcanic peaks, flood basalts form extensive gently sloping plains or plateaus. Shield volcanoes (discussed on the USGS web page) are also made up of many flows of relatively low-silica lava, but in this case the flows build up to form a large mountain like Mauna Kea in Hawaii (Figure 6).
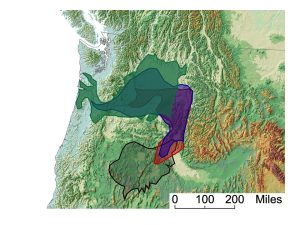
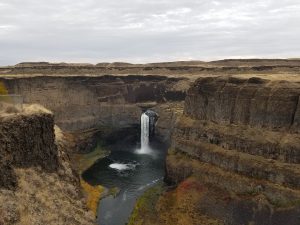
Another common product of volcanic eruptions is pyroclastic material, rock particles of various sizes that form when magma cools in air (another word often applied to these materials is tephra, used in the USGS web page). Pyroclastic material is especially associated with explosive eruptions, though it can form from magma with a range of silica content. The most widespread form of pyroclastic material is volcanic ash, produced when an explosive eruption shoots rock that is still cooling and solidifying high into the air in a large cloud (Figure 9). The result is a kind of dust that is blown by the wind over hundreds or even thousands of miles (Figure 10). Large amounts of volcanic ash are often erupted by composite volcanoes (see USGS web page).
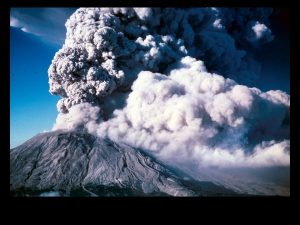
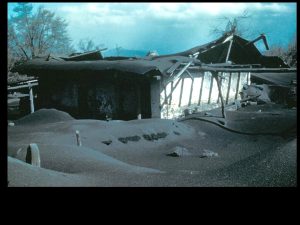
Larger pieces of pyroclastic material are occasionally carried through the air as well, like the volcanic bomb in Figure 11. Small volcanic cinders cover the slopes of cinder cones. Finally, many eruptions produce pyroclastic flows that travel down the sides of the volcano and through adjacent river valleys. Sometimes the material in these flows forms the very porous rock called pumice.
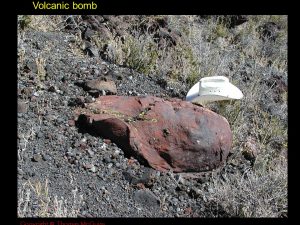
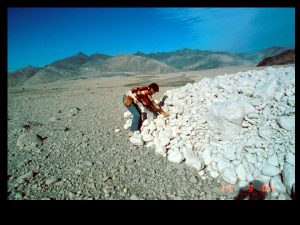
Types of Volcanoes and Silica Content of Magma
Types of volcanoes are discussed on the USGS web page linked above. The diagram in Figure 13 shows the most common association between volcano types and silica content of the magma that is erupting. Note that there is a lot of variation in silica content for each volcano type, however. For example, some composite volcanoes are produced by much higher silica magma than others. The term caldera, on the left side of Figure 13, is not defined on the USGS website. A caldera is a large crater produced by a highly explosive eruption (usually high-silica magma). In brief, the caldera forms after large volumes of pyroclastic material are erupted, and the land surface collapses into the space where that material came from. Crater Lake in Oregon, shown in Figure 14, is a relatively small caldera produced by an explosive eruption of a composite volcano thousands of years ago. There are other calderas in the western US, including the Yellowstone region, that are much larger–50 kilometers or more in diameter.
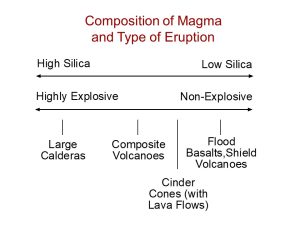
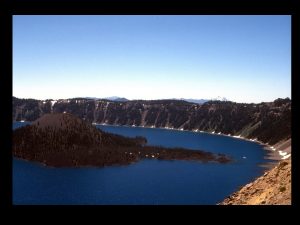
Location of Volcano Types Relative to Plate Tectonics
There are ongoing volcanic eruptions below the ocean at divergent plate boundaries all around the world, but this has little direct impact on humans. Many of the best-known volcanoes occur along convergent plate boundaries in subduction zones. These are mostly composite volcanoes. In the US, these include the volcanoes of the Cascade Mountains, like Mt. Rainier, Mt. St. Helens, Mt. Hood, and Mt. Shasta, along with many more in Alaska. Mt. Fuji and other composite volcanoes of Japan are along a subduction zone, as are the volcanoes of Indonesia and the Andes in South America.
One other plate tectonic setting for volcanoes still needs to be discussed. This is the hot spot, a location within a plate, often far from its boundaries, where there is frequent volcanic activity. Hot spots are related to the presence of mantle plumes, locations where large amounts of hot rock from deep in the Earth’s mantle flows upward toward the surface, producing repeated volcanic eruptions (Figure 15).
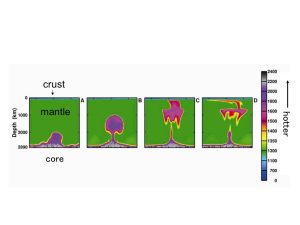
Mantle plumes remain fixed while tectonic plates move over them. Therefore, the key sign of a hot spot is a chain of volcanoes, showing past and present locations of volcanic activity over the mantle plume. The best-known example of a hot spot is now located under the Big Island of Hawaii. As the Pacific Plate moved over this hot spot, it produced earlier eruptions on the other islands of Hawaii, and before that on islands farther to the northwest that have now been eroded and collapsed so they are seamounts, mountains on the ocean floor that do not rise above the ocean surface.
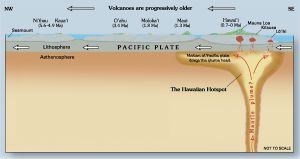
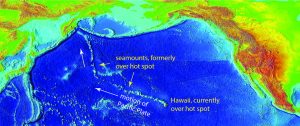
Volcanoes at the Hawaiian hot spot, on an oceanic plate, involve low-silica magma and are shield volcanoes. One explanation for the eruption of flood basalts in the Pacific Northwest of the US and other locations around the world is that these are also locations of mantle plumes, erupting low-silica mafic magma (not all geologists agree with this interpretation). Most geologists agree that there is a hot spot now located under Yellowstone National Park, which has produced a series of highly explosive eruptions of high-silica magma, resulting in huge calderas. These are among the largest volcanic eruptions in recent geologic history (last several million years). There have also been large explosive caldera eruptions in other parts of the western US, which are not related to hot spots and have a more complex plate tectonic explanation.
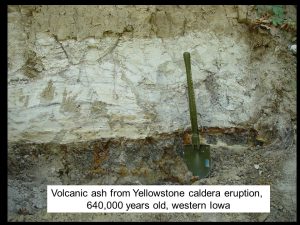