Lab 2 Background
Amplify the T7 Mutant Phage Genome
As discussed in Chapter 1, the T7 phage is an obligatory lytic phage that infects most E. Coli strains. To understand the mechanism of T7 phage infection and to study the virulence of the T7 phage, each pod will construct and test one T7 mutant. The wild-type, unaltered T7 phage will be used as a baseline control for comparison.
To successfully construct and express a T7 mutant, we will do the following steps in today’s lab:
- Use PCR to fragment the T7 genome and introduce the desired mutation:
- The genome of the T7 phage is about 50 kilo-base pairs (kb), or 50,000 base pairs (bp), a considerably long stretch of DNA. To successfully express the T7 phage, we will break down its genome into five pieces using PCR.
- This means that we will make five different PCR reactions with the same DNA template (the T7 genome) and five different sets of primers (PCR and primer are explained below).
- This allows us to obtain DNA fragments with manageable lengths for later genome assembly and expression. The PCR process also allows us to introduce the desired mutation into the T7 phage genome by making copies of the genome fragments with the mutation.
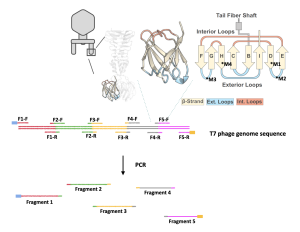
- Use PCR to fragment the expressing vector pRS315:
- A vector, or plasmid, is an artificial circular extrachromosomal DNA where genes can be inserted and subsequently expressed in a desired organism.
- In this research project, we will use the vector pRS315.
- This vector is used because it has several important features:
- It contains a type of DNA called yeast artificial chromosome, which allows the T7 phage genome to be reassembled in yeast cells.
- It can carry and deliver the mutant T7 phage genome (constructed by you) into bacteria.
- It has features that allow for proper T7 phage gene expression in the bacteria, which will lead to phage particle production and host lysis/killing.
- Similar to the T7 phage genome, we will also break up the DNA sequence of the pRS315 vector into 3 fragments using PCR.
- This means that we will make three different PCR reactions with the same DNA template (pRS315) and three different sets of primers.
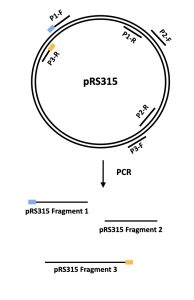
Polymerase chain reaction (PCR)
PCR is a laboratory adaptation of DNA replication that uses polymerase to rapidly make millions and billions of copies of DNA. It can be used to amplify an entire DNA region, but it is more often used to amplify a segment of the DNA that the experimenter is interested in. For instance, if the experimenter is interested in studying gene A on chromosome X. Instead of amplifying the entire chromosome X, the experimenter can use PCR to amplify gene A only. In addition to making copies of DNA, PCR can be used to introduce mutations in DNA by introducing a mutation in the primers, which are short strands of DNA that are needed for PCR. Mutations can be introduced using primers because primers will become part of the newly synthesized DNA.
- Phusion Polymerase: in our laboratory, we will use Phusion polymerase, a heat-resistant enzyme that can recognize DNA and use nucleotides, the building blocks of DNA, to make copies of the DNA template. The Phusion enzyme is a fast-action enzyme that can make 1kb of DNA in about 15 seconds. To make copies, the Phusion polymerase needs a starting point, which is provided by the primers.
- Primers: primers are short pieces of single-stranded DNA designed by the experimenter that is at least partially complementary to the DNA template. By binding to the DNA template, the primers define the location of the amplification. If the experimenter wants to amplify gene A, for example, the experimenter will design two primers, one binding to the very beginning of gene A and the other binds to the end of the gene. Once the primers bind to the DNA template and act as a starting point for the Phusion polymerase, the polymerase can fill in the remaining gene sequence and make a copy of the gene. If a mutation is desired, one can design primers that are slightly different from the template DNA sequence. If the difference is not much, the primers still bind to the DNA template, and the final product, which begins with the primer sequence, will contain the mutation.
Agarose Gel Electrophoresis
Agarose gel electrophoresis is a technique that uses electrical current to separate nucleic acids based on size or shape. Agarose, a main component of agar, forms a porous gel that nucleic acids can migrate through when the current is applied. Because nucleic acids are negatively charged, they can migrate from the negatively charged side of the current to the positively charged side during the electrophoresis. This separates the nucleic acids by size, with the smallest nucleic acids migrating faster through the pores and running further down the gel.

Different concentrations of agarose are used in gels depending on the sizes of the nucleic acids: the larger the nucleic acid, the lower concentration of agarose. For technical reasons, agarose gels generally range from 0.5% to 2.5% weight by volume (W/V). In this experiment, you will run a 1% agarose gel.

In this laboratory, your PCR DNA samples will be mixed with a 6X loading dye (1 μL of loading dye per 5 μL sample) containing tracking dyes and sucrose. While the dense sucrose helps the samples to sink to the bottom of the wells, the tracking dyes function as trackers of the sample migration during gel running.
Reference
Brunstein, J. (2013). PCR: the basics of the polymerase chain reaction. MLO Med Lab Obs, 45(4), 32, 34-35. Retrieved from https://www.ncbi.nlm.nih.gov/pubmed/23822023
Huss, P., Meger, A., Leander, M., Nishikawa, K., & Raman, S. (2021). Mapping the functional landscape of the receptor binding domain of T7 bacteriophage by deep mutational scanning. Elife, 10. doi:10.7554/eLife.63775
An enzyme which brings about the formation of a particular polymer, especially DNA or RNA.
Short pieces of single-stranded DNA designed by the experimenter that is at least partially complementary to the DNA template.