Neuronal Mechanisms for Information Transmission
How information is transmitted between neurons | Synaptic transmission at chemical synapses
Structure of the synapse
Axons have terminal swellings called boutons, which contain synaptic vesicles. Synaptic vesicles contain the chemical signal in the form of molecules called neurotransmitters or transmitters. They are called transmitters because they transmit the neural signal from the signaling cell, which is called the presynaptic cell, to the receiving cell, which is called the postsynaptic cell. The narrow space between the membrane of the signaling cell and the membrane of the receiving cell is called the synaptic cleft (20 nm). Together, the presynaptic membrane, the synaptic cleft, and the postsynaptic membrane are called the synapse.
Watch: Synaptic Transmission – Narrated Animation (3:37)
Neurotransmitter release: sequence of events
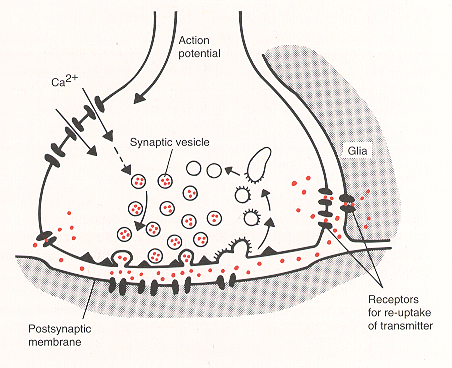
- Action potential invades presynaptic terminal
- Depolarization opens Ca2+ channels
- Increased intracellular Ca2+ concentration mediates release of neurotransmitter via exocytosis of synaptic vesicles
- Neurotransmitter diffuses through the synaptic cleft and combines with receptors on the postsynaptic membrane
- Changes in membrane potential of the postsynaptic membrane: post-synaptic potentials (PSPs)
- Reuptake or degradation of neurotransmitter
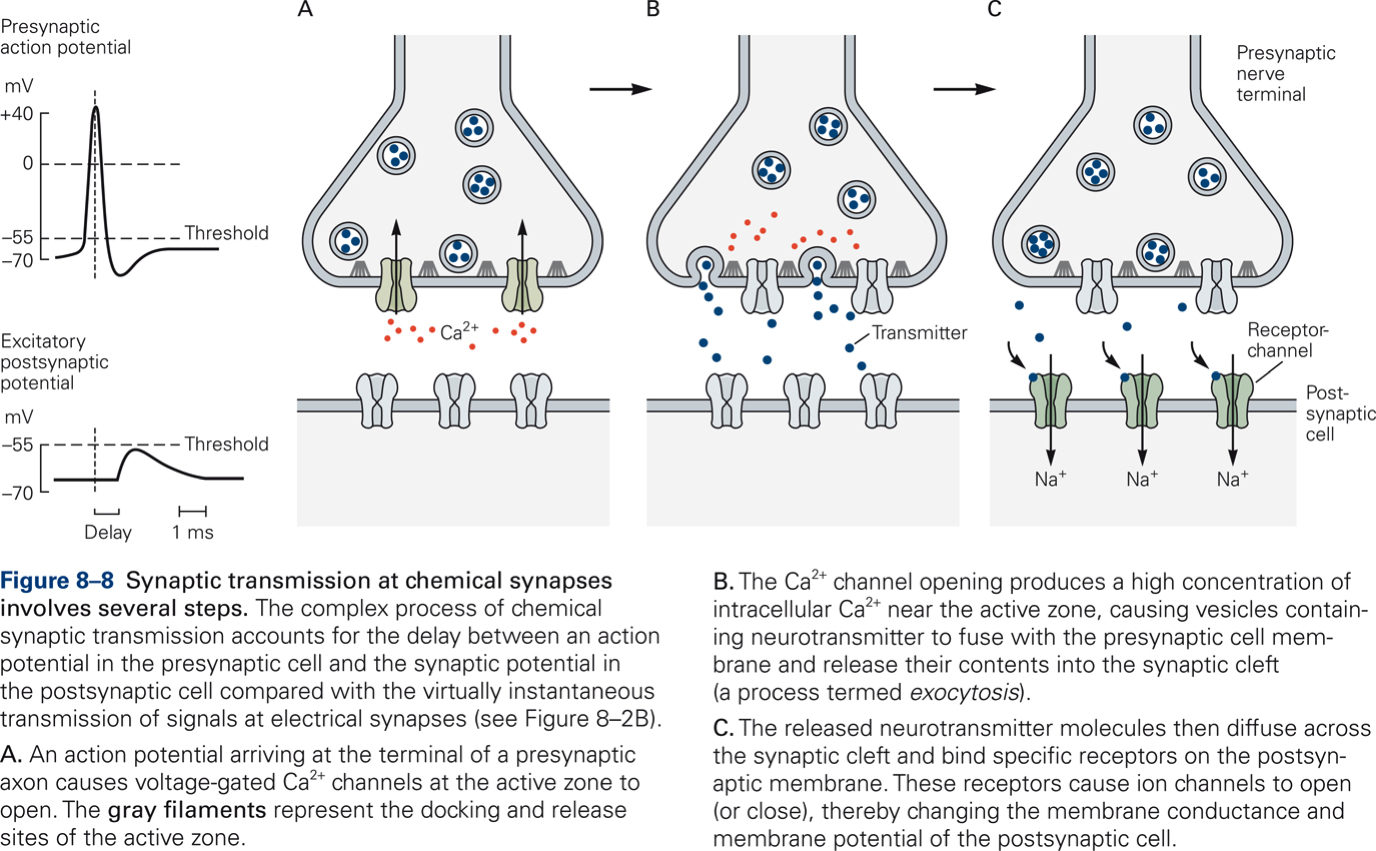
Synaptic Potentials
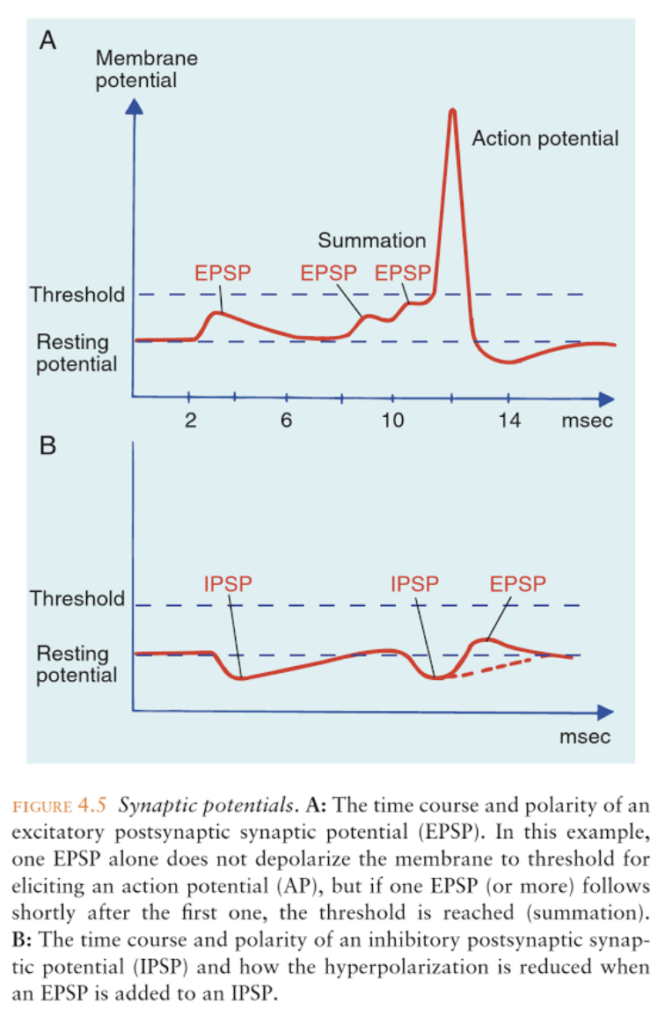
Excitatory post-synaptic potential (EPSP)
- Neurotransmitter increases permeability for Na+
- Depolarization: Excitatory PostSynaptic Potential (EPSP)
- EPSP brings membrane potential closer to threshold
Inhibitory post-synaptic potential (IPSP)
- Neurotransmitter increases permeability for K+ or Cl-
- Hyperpolarization: Inhibitory PostSynaptic Potential (IPSP)
- IPSP brings membrane potential further away from threshold
Postsynaptic receptors
Postsynaptic receptors, not neurotransmitter, determine whether a PSP is excitatory or inhibitory. All postsynaptic receptors influence the opening and closing of ion channels. All postsynaptic receptors are membrane-spanning proteins.
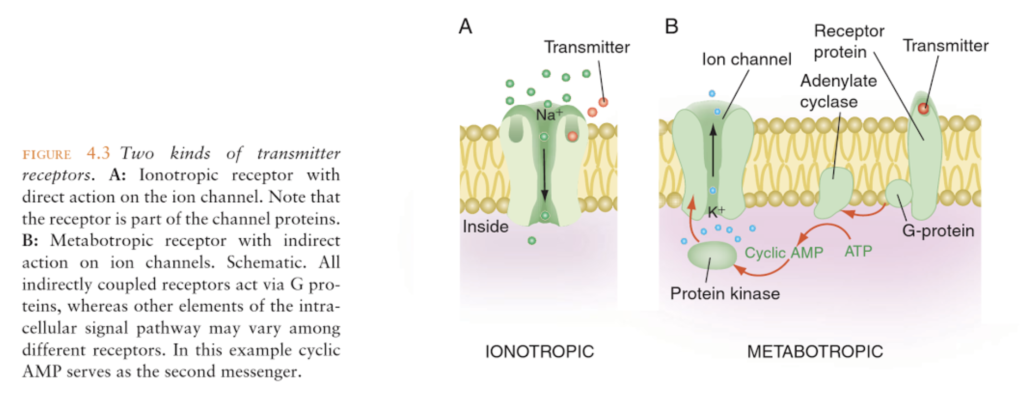
Receptors that gate ion channels directly (ionotropic)
- Structurally part of the ion channel
- Neurotransmitter affects ion channel directly
- Fast PSPs (range: few ms)
- Function in circuits that directly mediate behavior
Receptors that gate ion channels indirectly (metabotropic)
- Spatially separate from ion channels
- Neurotransmitter acts indirectly via second messengers (e.g. cAMP)
- Slow PSPs (range: seconds or minutes)
- Neuromodulatory (e.g. involved in learning)
Neurotransmitters
Criteria
For a molecule to be considered a neurotransmitter, it has to fullfil the following criteria:
- Synthesized in neuron
- Present in presynaptic terminal
- When applied to synapse experimentally, it mimics effects of transmitter exactly
- Specific mechanism exists to remove it from synaptic cleft
Classification
There are two broad classes of neurotransmitters: small molecule and large molecule transmitters.
Small molecule transmitters
Amino acid transmitters
- Major excitatory transmitter: GLU
- Major inhibitory transmitters: GABA, GLY
Acetylcholine (ACH)
- Fast synaptic effects
- Neuromuscular junction
- Mainly excitatory
Monoamines (Biogenic amines)
- Catecholamines (NE, DA)
- Histamine and serotonin
- Fast and slow effects
Large molecule transmitters: Neuropeptides
- Slow inhibitory or excitatory effects
- Co-localized with small molecule transmitters
Neuromuscular junction
Key Takeaways
Synaptic transmission at neuromuscular is simpler than that at synapse between two neurons because:
- Muscle fibers innervated by only one motor neuron
- Muscle fibers receive only excitatory input
- All synaptic potentials mediated by ACH
- Each synaptic potential produces an action potential
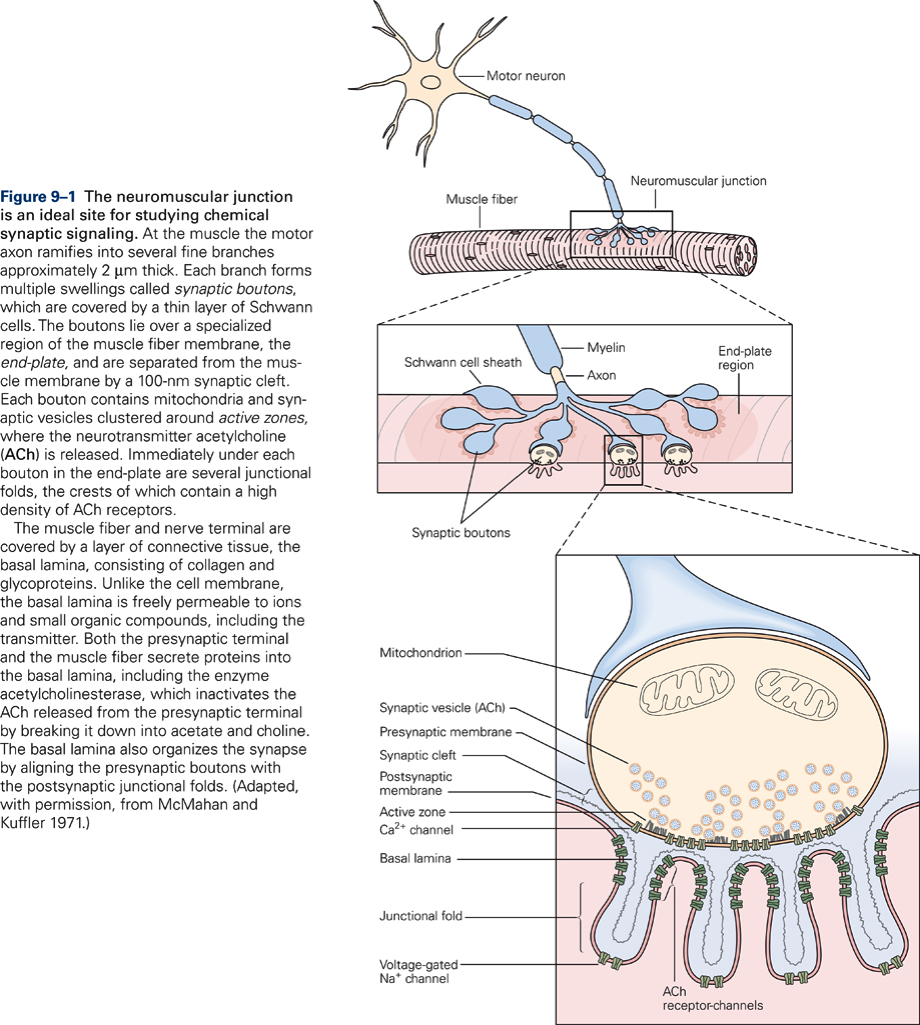
Electrochemical signals involved in encoding stretch of a muscle
![Diagram of the characteristic signals of the neuron's four signaling components. [Citation]](https://wisc.pb.unizin.org/app/uploads/sites/202/2018/01/KINES200_M1_10_neuronsignalling.png)
The trigger zone of the muscle receptor (muscle spindle) (Fig. 2-10B) integrates the input signal (i.e. the receptor potential) and produces action potentials that will be propagated along the axon. An action potential is generated only if the input signal is greater than a certain spike threshold. Once the input signal exceeds threshold, any further increase in amplitude of the input signal increases the frequency with which the action potentials are generated, not their amplitude. The frequency and number of action potentials generated depends on the amplitude of the receptor potential and the length of time the receptor potential exceeds threshold. The conversion of stimulus amplitude into frequency of action potentials is called frequency coding. Frequency coding is the major way information is communicated in the CNS.
The information in the signal is represented only by the frequency and number of action potentials, not by their amplitude. If a stimulus is strong enough to bring the membrane to threshold at the trigger zone, an action potential starts and travels the entire length of the axon in an all-or-none fashion (analogous to pushing the lever to flush the toilet), and at a self-sustaining, constant amplitude (Fig. 2-10C). When the action potential reaches the output component of the neuron (the synaptic terminal, Fig. 2-10D), the neuron releases a chemical neurotransmitter, which is the output signal. The total number of action potentials in a given time period specifies exactly how much neurotransmitter the neuron will release. Neurotransmitter molecules transmit the signal from the signaling neuron (the presynaptic cell) to the receiving neuron (the postsynaptic cell). Neurotransmitter molecules diffuse in the space between pre- and postsynaptic cells (the synapse) and combine with receptors on the input component of the postsynaptic cell. The interaction between transmitter and receptors generates a graded potential in the postsynaptic cell that is qualitatively similar to a receptor potential but is referred to as a synaptic potential.