Spinal Mechanisms for Sensorimotor Integration
The Somatosensory System
No other sense can arouse you like touch. . . . If touch didn’t feel good, there’d be no species, parenthood, or survival. . . .touch is not only most basic to our species, but the key to it.
—The late Duke neuroscientist Saul Schanberg, quoted by science writer Diane Ackerman in her book A Natural History of the Senses (1990)
The sense of touch is fundamental to human existence. Touch orients us to our internal and external worlds by providing the interface through which we experience what we are sensing. Tactile sensors not only provide information about size, form, orientation, and texture of objects in our environment needed for their recognition and manipulation, but touch also informs human social interactions, emotional responsiveness, and social bonding. We can readily envision and experience life without vision, audition, or olfaction; however, envisioning life without somatosensation is virtually impossible.
The importance of the sense of touch for both verbal and non-verbal communication cannot be overstated. Touch permeates our everyday lives so deeply and profoundly that we are often unaware that many words and expressions we use in everyday language find their origin in the sense of touch. For example, in order to express emotional involvement, such as sympathy or empathy, we speak of how something or someone ‘touched us deeply,’ or we characterize a difficult problem as ‘hard’ or a precarious situation as ‘touchy.’
General principles of organization
Our tactile experience relies on many types of sensory receptors in the skin and muscles, each specialized to extract information about different aspects of our tactile world. Sensors in the skin that provide information about touch, pressure, vibration and stretch are distinct from those that monitor temperature and convey responses to painful stimuli, which are distinct from the sensors that are activated by a sensual caress. The nerve fibers that transmit the different types of information to the spinal cord are largely devoted to a single class of somatosensation. As the information is transmitted from the skin to nerves to brain, the neural signals that encode the different types of tactile information are blended and combined with signals from other sensory modalities. Touch signals are integrated with proprioceptive signals, which convey the state of contraction of muscles and positions of joints, with vestibular signals, which conveys the orientation of the body in space, and with visual and auditory signals, which are essential for mapping extra personal space. At the highest levels of central processing, sensorimotor circuits interact with neural circuits that underlie emotion, motivation, and higher cognitive functions, and it is this multimodal blending of neural signals that provides us with a rich, useful, and unitary percept.
Adaptation
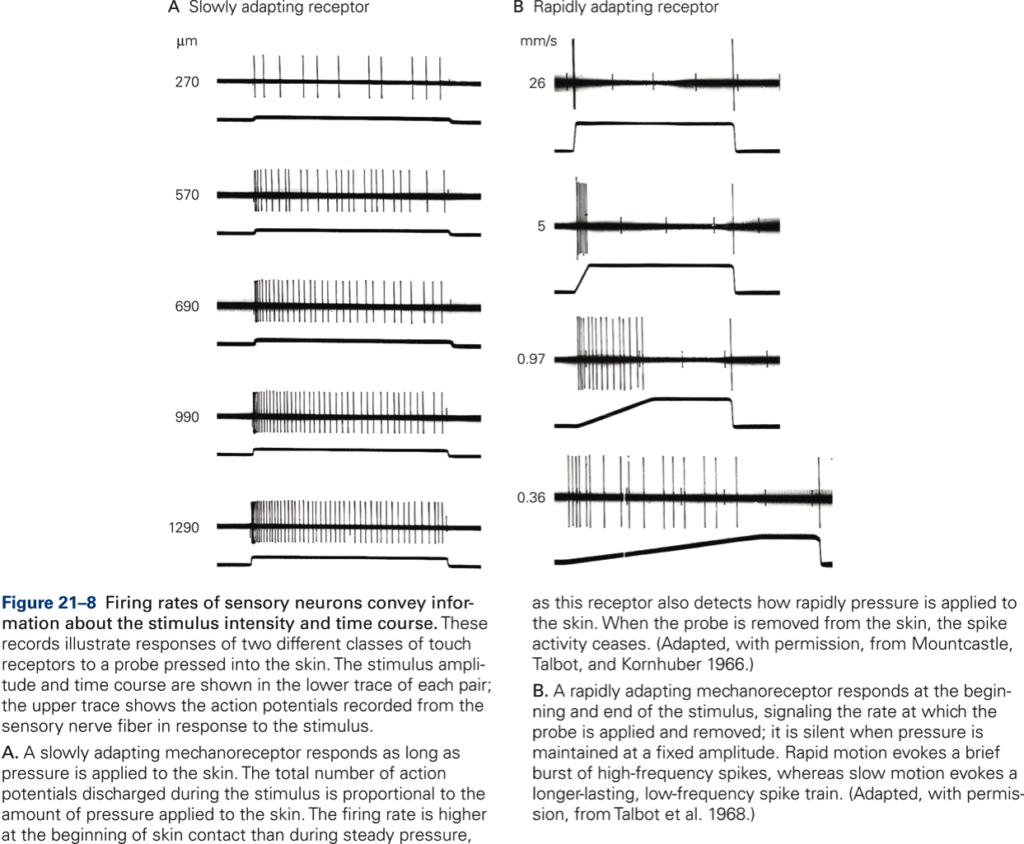
The phenomenon that receptor potentials and the firing rate of a sensory neuron decline over time while a stimulus of constant intensity is applied to the receptor is referred to as receptor or sensory adaptation. Receptor adaptation may be the neural substrate for perceptual adaptation that occurs when a constant stimulus fades from consciousness over time. Adaptation is not due to fatigue but is an inherent property of the sensory receptor. If the decline in receptor potential and firing rate is slow, the sensory receptor is called a slowly adapting receptor. If it is fast, it is called a rapidly adapting receptor. A slowly adapting receptor starts at the onset of stimulation, and the response declines slowly, i.e. it is maintained throughout the period of stimulation. Slowly adapting mechanoreceptors therefore can provide information about the intensity of stimulation. In contrast, rapidly adapting receptors respond solely at the beginning or end of the period of stimulation; they cease firing while the stimulus is maintained, so no information is conveyed about the intensity of the stimulation. Rapidly adapting receptors do not respond to the sustained stimulus strength but to the rate at which the stimulus strength changes. For example, Fig. 21-8B shows the pattern of firing of a rapidly adapting mechanoreceptor to skin indentations at three different, constant speeds (measured in millimeters of skin indentation per second, mm/s). In each case, the afferent firing rate depends on the speed of skin indentation, not indentation per se.
Receptive field
The location and spatial aspects of a stimulus such as the size, shape, and orientation of an object touching the skin are encoded by the location of receptors in the sense organ, and the size and density of their receptive fields. The receptive field is defined as the area of skin from which a sensory neuron may be activated.
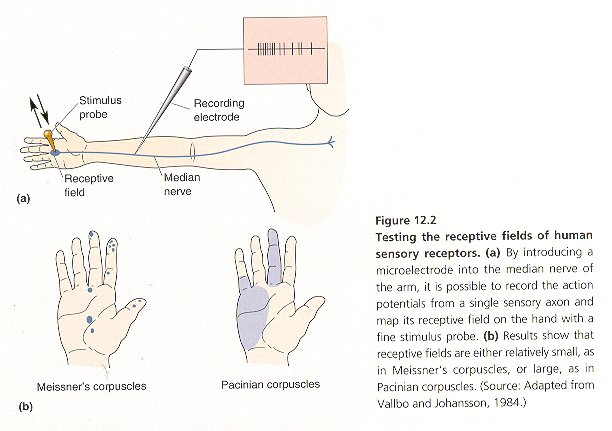
Receptive fields of human sensory receptors can be investigated with electrophysiological techniques (Fig. 12-2). By introducing a microelectrode into the median nerve of the arm, it is possible to record the action potentials from a single sensory axon and map its receptive field on the hand with a fine stimulus probe (Fig. 12-2A). Receptive field sizes vary over a wide range, from relative small, as in Meissner’s corpuscles, to large, as in Pacinian corpuscles (Fig. 12-2B).
The spatial resolution of a sensory system is proportional to the total number of receptor neurons and the size and density of their receptive fields. Tactile acuity is highest on the tips of the fingers because here the density of receptors is highest and receptive fields are small. Each sensory neuron detects stimuli in a small area of skin, but because there are so many of them, the population of neurons in a fingertip transmits a very detailed representation of the stimulation. Tactile acuity can be quantified by measuring two-point discrimination thresholds, defined as the minimum distance at which two stimuli applied at nearby sites on the body surface are resolved as distinct. Two-point discrimination thresholds vary with location on the body surface – thresholds are lowest at the fingertips and greatest on the calf, upper arm, and back.
Classification of sensory receptors
The mechanisms that underlie the generation of receptor potentials differ for different types of receptor – the mechanisms of light transduction in photoreceptors is different from the mechanisms of chemical transduction in taste and olfactory receptors (chemoreceptors), the mechanical transduction in touch, muscle, and vestibular receptors (mechanoreceptors), and the transduction of thermal energy in thermoreceptors – however, in all cases, stimulation of the receptors is transduced into receptor potentials. Receptor potentials are then converted into action potentials in primary afferent (sensory) axons that transmit information from the receptor cells to the central nervous system. Stimulation of sensory receptors provides the information needed for sensory perception and goal-directed motor activity.
Sherrington first classified sensory receptors based on the origin of the stimulation into exteroceptors, interoceptors, and proprioceptors. Exteroceptors respond to mechanical, thermal, chemical, or electromagnetic energy in the external environment. Examples of exteroceptors are mechanoreceptors in the skin sensitive to touch, pressure, or stretch, photoreceptors in the eye, and chemoreceptors in the brain’s olfactory bulbs. Interoceptors respond to stimulation that results from physiological processes within the body. Examples of interoceptors are thermoreceptors in the deep tissues that are sensitive to internal temperature of the body, mechanoreceptors in the walls of blood vessels and intestines, and chemoreceptors in internal organs. Proprioceptors are sensitive to mechanical stimulation associated with the state of contraction of muscles, movement and angular position of joints and ligaments, and the orientation of body segments.
Neural encoding of sensory information
All sensory systems encode four elementary attributes of stimuli: modality, location, intensity, and time course. Modality refers to the different classes of sensations we are capable of responding to, such as vision, audition, taste, smell, and somatosensation. Modalities are further subdivided into sub modalities, also referred to as qualities. For example, temperature, rigidity, and texture are qualities of touch, bitter is a quality of taste, color is a quality of sight, etc. The location and spatial characteristics of a stimulus are associated with the spatial distribution of the population of activated receptors and their receptive fields. The intensity and duration of stimulation are reflected in the amplitude of the receptor potentials and the number of receptors activated. More intense stimuli give rise to higher frequencies of neuronal firing. Temporal features of stimuli are represented in the time course of firing action potentials.
Sensory receptors are selective: each sensation is mediated by specialized receptors capable of transducing specific forms of energy into neural signals. For example, activation of photoreceptors in the retina elicits visual sensations and activation of distinct types of mechanoreceptors in the skin elicits specific tactile sensations. We say that a given receptor is ‘tuned’ to an optimal or preferred stimulus. The adequate stimulus is defined as the stimulus type that requires the least amount of energy to produce a response. A second factor in achieving selectivity of receptors is by placement and shielding of receptors in such a way that activation of the receptor by non-specific stimuli is prevented or minimized. Under natural conditions, it is highly unlikely that a receptor is sensitive to stimulation of a modality other than the modality for which the receptor is selective. Thus, it follows that the response of a given receptor type carries information about a specific type of stimulation. This concept is referred to as a labeled line code: the pathway along which signals that originate from a given receptor type are transmitted is the ‘line’, and the line is ‘labeled’ in that it derives from activation of a specific receptor type.
Sensory systems provide us with information necessary for producing goal-directed behavior. We obtain information about the external world by processing and integrating extended spatiotemporal patterns of stimulation of large numbers of receptors. The process of obtaining information about our surroundings and the body from sensory stimulation and making the information available for decision making, planning and controlling motor actions, reasoning, memorizing and communicating is referred to as sensory perception. Perception requires processing of receptor responses; it is an active process that involves motor behavior. Obtaining tactile information is called feeling and requires actively moving the hand and fingers over objects. Obtaining visual, auditory, and olfactory information is called looking, listening, and smelling (or sniffing), respectively, and similarly requires active movements of various body parts. Thus, perception is not only essential for motor behavior; motor behavior is also essential for perception. In fact, the sensitivity of some sensory receptors is regulated by efferent (motor) signals. For example, a special class of motor neurons (gamma motor neurons) can adjust the sensitivity of muscle receptors (muscle spindles) that measure muscle length and muscle stretch.
Many mechanoreceptors provide somatosensory information that is modality specific, that is, the information conveyed is distinct for different types of somatosensory receptors. For example, cutaneous mechanoreceptors, which underlie our ability to recognize objects by touch, respond to specific mechanical deformations of the skin, sensing touch, pressure, vibration, or stretch. Golgi tendon organs in our muscles respond to changes in the force of muscle contraction, which enable us to regulate contractile forces over a wide dynamic range, from a barely perceptible muscle twitch to a contraction so powerful that it results in injury. Muscle spindles respond to changes in muscle length, thereby providing proprioceptive information that underlies our sense of body and limb position, which is critical for manual dexterity and coordination of movement in general. Nociceptors produce pain sensations that protect our bodies from injury.
Perception, however, is multimodal. Somatosensory information interacts with and is integrated with information from other sensory modalities, such as vision and audition, to produce a single, integrated experience. The concept of cross-modality, or multisensory integration, is a key principle in perceptual neuroscience and psychology that is typically difficult to grasp and to some extent counterintuitive because in our everyday subjective experience sensory modalities appear separate: we smell, we touch, we see, we hear, and we typically are not aware that these seemingly distinct experiences are in reality fully integrated with one another: our perceptual experience is unitary. The rubber hand illusion is an example of a visually induced somatosensory percept. The McGurk effect is another example of multisensory integration, in this case, involving vision and audition.
Classification of somatosensory sensations
There are four classes of somatosensory sensations: 1) Touch, which includes sensations of contact, pressure, movement, stroking, and vibration. Touch is used to identify objects and can include an active motor component, pressing, stroking, grasping, tapping, whereby one body part is moved over another surface or another being. Sensory and motor components of the neural circuits underlying touch are intimately connected with one another and are essential for guiding action. 2) Conscious awareness of the position and movement of one’s own body (kinesthesia or conscious proprioception), provided by receptors in muscles, ligaments, joint capsules, and skin. 3) Sensation of temperature, heat and cold, provided by thermoreceptors. 4) Nociception, the sense of pain, which is a response to tissue injury. Stimulation of thermoreceptors and nociceptors can elicit skeletomotor and autonomic involuntary reflexive movement, such as the withdrawal of a hand touching a hot surface or producing goose pimples in cold weather.
A feature common to all somatosensory receptors is that they convey their information by dorsal root ganglia neurons that innervate the trunk and limbs or by trigeminal sensory neurons that innervate cranial structures, the face, lips, and the head. The cell body of a dorsal root ganglion neuron lies in the dorsal root of a spinal or cranial nerve. The axon of a dorsal root ganglion cell bifurcates (Fig. 2-10). One branch projects to the periphery and innervates the skin, muscles, joint capsules or viscera; the other branch projects to the central nervous system. Thus, the axon represents a direct line for the transmission of somatosensory information from peripheral receptors to the CNS and, therefore, is referred to as a primary sensory afferent fiber. Axons bundle together in fascicles to form spinal or cranial nerves.
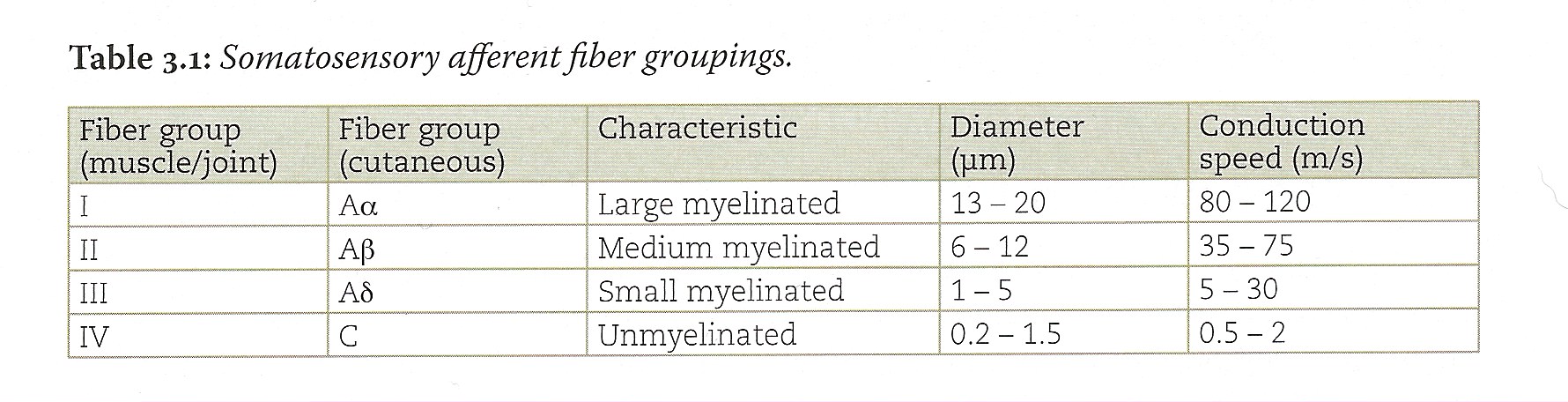
Primary sensory afferent fibers of different modality differ in their diameter and myelination; therefore, they conduct action potentials at different speeds. Some primary sensory afferent fibers are myelinated; some are not. Axon diameter varies over a large range, from 0.2 μm for the thinnest (unmyelinated) fibers to 20 μm for the large myelinated fibers. Mechanoreceptors for touch and proprioception are innervated by myelinated sensory afferents of large diameter that conduct action potentials rapidly. Thermal receptors, nociceptors, and the low threshold mechanoreceptive C tactile (CT) afferents, which convey affective (pleasant) touch, have small diameter axons that are only thinly myelinated or unmyelinated and conduct action potentials more slowly.
Sensory afferent fibers are classified in four categories (I-IV) based on myelination and fiber diameter (Table 3-1). An alternative nomenclature is alphabetical and is based on conduction speed. Because there is a simple one-to-one relation between axon diameter and conduction speed, the two nomenclatures are equivalent. The alphabetical nomenclature is usually used for cutaneous whereas the numerical naming is used to describe receptors that innervate muscle and joint receptors. Somatosensory mechanoreceptors comprise the so-called large-fibered afferent system as their fibers are of group I (Aα) and group II (Aβ) varieties. Nociceptors, thermoreceptors, and C tactile afferents comprise the small-fibered afferent system; their fibers are group III and IV (Aδ and C, respectively).