4 How does Nitrogenase use ATP to drive Nitrogen Fixation?
We’ll first examine the ATP mystery and to do so we’ll begin by looking at the Fe protein cycle (Fig. 4.1).
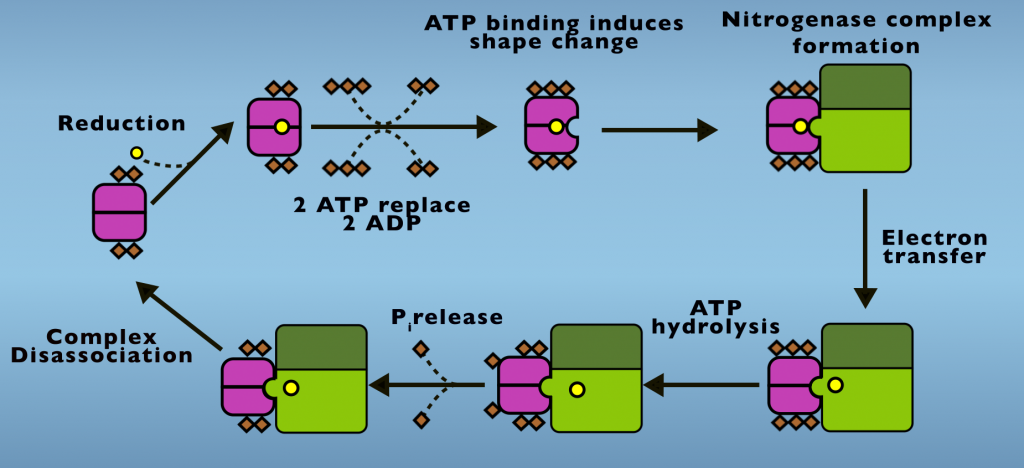
In its initial condition two ADP are bound to the Fe Protein and the F-cluster is in an oxidized state. From this point, a protein that carries electrons in cells, something called either a flavodoxin or ferredoxin transfers one electron to the F-cluster. Next, two ATP displace two ADP from the reduced protein and ATP binding initiates a structural change in the Fe Protein. This is also called a conformational change. This is the first energetic input of ATP into Nitrogenase activity. The conformational change caused by ATP binding enables the iron protein to dock with the MoFe Protein, creating the Nitrogenase complex. Electron transfer occurs following complex formation.
The electron transfer step is actually a process onto itself. Which we’ll return to examine in more detail, shortly. ATP hydrolysis follows electron transfer and both the products of ATP hydrolysis, ADP and phosphate, remain bound to the Fe Protein. Phosphate is released in a subsequent step and it’s the release of phosphate that allows the complex to disassociate.
Phosphate release is the slowest step of the Fe Protein Cycle and is the step that limits the rate at which Nitrogenase carries out in Nitrogen Fixation. Following phosphate release, the Fe Protein and MoFe Protein return to their initial states. This cycle repeats until eight electrons are transferred and N2 reduction is completed.
Now to gain a deeper understanding of the role of ATP in driving nitrogen fixation, we’ll examine in more detail the electron transfer process that occurs in the Nitrogenase complex. There are actually two electron transfer events that occur sequentially (Fig. 4.2).
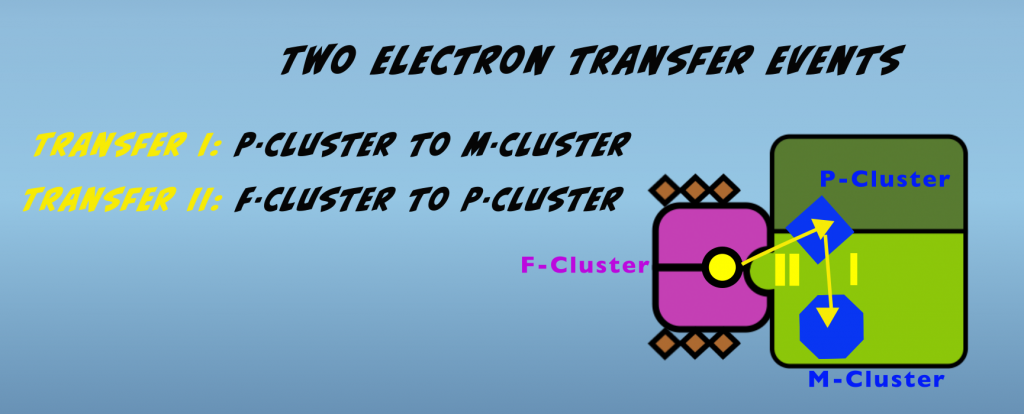
The first occurs within the molybdenum iron protein and is a transfer from the P-cluster to the M-cluster. This is followed by electron transfer from the F-cluster of the iron protein to the P-cluster of the molybdenum iron protein. We’ll look at each of these in turn.
Transfer one is initiated by the binding of the iron protein to the molybdenum iron protein. This binding causes conformational changes in the molybdenum iron protein that enable an electron to transfer from the P-cluster to the M-cluster. The exact nature of these alterations is unknown, but they are hypothesized to be rearrangements of amino acids, which have the effect of opening a gate that allows electron passage. This hypothesized mechanism is thus termed “Conformational Gating”. A related concept that applies to this first electron transfer is called “Deficit Spending.” This refers to the P-cluster transferring or “spending” an electron prior to receiving one from the iron protein.
The second electron transfer is also supported by conformational changes that are induced by complex formation. In this case, the alteration occurs in the structure of the iron protein and increases the energy state of the F-cluster. The graph below (Fig. 4.3) illustrates this important, energetic impact.
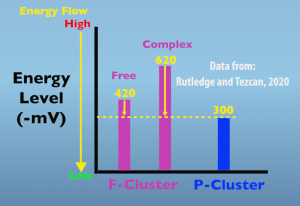
In the free, non-complexed, iron protein the F-cluster energy level is 420 millivolts, which is increased to 620 millivolts when the in protein is in the complex. The key thing to remember is that energy naturally flows from higher to lower states. In this case, energy is in the form of an electron and is moving from the F cluster to the P cluster. The functional significance of this elevated energy level is that it greatly increases the amount of energy driving electron transfer from the F cluster to the peak cluster.
Prior to electron transfer, it’s hypothesized that the iron protein migrates from its initial docking site to the interface of the alpha and beta subunits (Fig. 4.4).
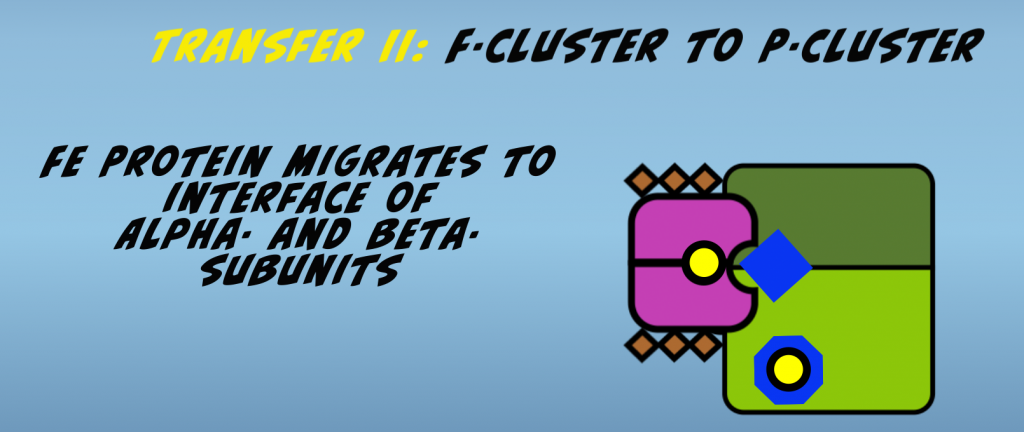
This facilitates electron transfer by bringing the F-cluster and P-cluster in close proximity. The second electron transfer then occurs. The cumulative effect of the conformational changes is that the Nitrogenase complex is brought to an elevated energy state.
This energy must be released in order for the proteins comprising the complex to return to their baseline condition and be available for continued function in nitrogen fixation. This energy release is accomplished in two steps, starting with ATP hydrolysis, which is then followed by release of the phosphate in its own reaction.
It’s notable that phosphate release is needed to enable the complex to fully relax and for the two proteins to disassociate. Once dissociation occurs, the proteins are back to the baseline energy state and can begin another round of electron transfer.