5 Why does Nitrogenase produce hydrogen gas?
Now we’ll turn our attention to the mystery of H2 production by Nitrogenase. And to explore that subject, we’ll look at activities occurring within the MoFe Protein. We’ll begin with a broad overview of the MoFe Protein function, which is represented by the Lowe Thorneley kinetic model, or for short, the LT model. (Fig. 5.1). This model describes a series of intermediates formed by the MoFe Protein, as it progresses through each of its eight electron transfer interactions with the Fe Protein and integrates these with the processes of N2 reduction and H2 formation.
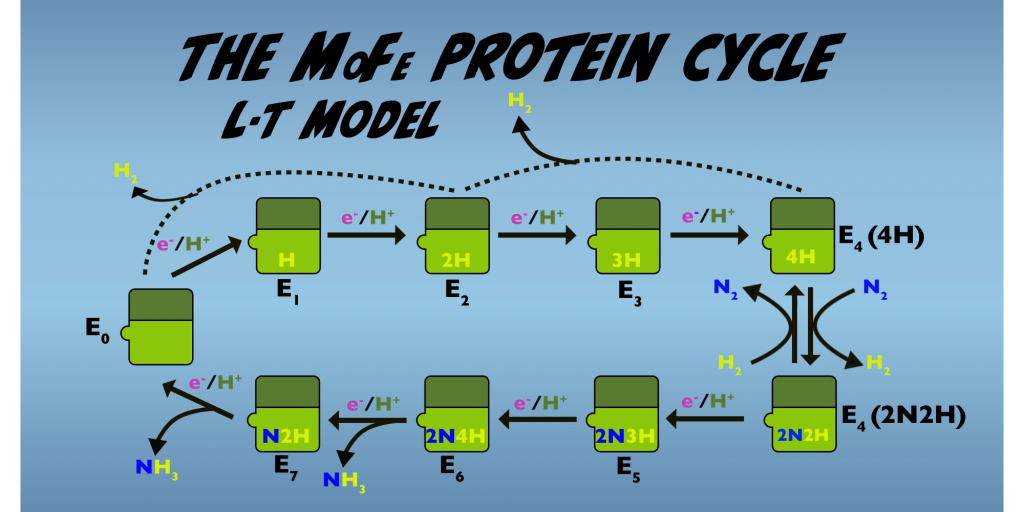
The starting point, also called the resting state, is “E0”. From this point, with successive electron transfer steps, the MoFe Protein passes through E1 to E4. At each stage, the addition of one electron and one proton enable formation of one Hydrogen atom. Note that none of the first four electron transfers directly involved in N2 reduction. Instead, these initial electron transfers and the eight ATP that have been consumed, contribute to the transformation of the MoFe Protein to the E4 state that has activated for N2 binding and reduction.
It’s the E4 state that we get the first insight into how Hydrogen gas production fits into Nitrogen Fixation. Here, the LT model holds that formation of one molecule of H2 occurs as a result of the binding of one molecule of N2. This process is reversible and bound nitrogen can be displaced by H2. But under ambient conditions where N2 levels are high relative to H2, the forward reaction is favored. The reactions occurring at E4 hold the key to solving the mystery of why H2 production is an essential characteristic of nitrogenous activity. We’ll return to examine E4 in more detail and delve into the mechanisms by which H2 is produced.
The MoFe Protein cycle can also run in reverse from E4. In this case, the MoFe Protein, releases Hydrogen gas and reverts to the earlier enzyme states; from E4 to E2 and potentially from E2 to E0. This reverse process is termed “relaxation” and is potentially a significant source of H2 . Hydrogen gas generation from relaxation is termed “unproductive H2 formation”, as it contributes, nothing to Nitrogen Fixation. This is in contrast with H2 formation associated with N2 binding, which is essential to the mechanism Nitrogenase employs for nitrogen fixation and is thus termed “productive H2 formation.”
Returning to the forward reaction. The details of bond breakage and hydrogen addition are not yet unambiguously established. Two general pathways have been postulated by which this initial nitrogen reduction and subsequent reductions occur. This presentation will follow one of these proposed pathways, which appears to be best supported by current research, which has termed the “Alternating Pathway”. In this pathway, the two hydrogen remaining in the molybdenum iron protein are immediately added to the bound Nitrogen, one to each of the Nitrogen atoms. In subsequent steps, electrons received from the Fe Protein are added in an alternating fashion to each of the bound nitrogen atoms. Hence the term “alternating”. In this pathway the two nitrogen atoms aren’t fully reduced and released as ammonia until the last two electron transfers.
We’ll now we return to E4 for a closer look and examine the mechanisms that tie hydrogen gas production to nitrogen fixation by Nitrogenase. The E4 intermediate has been termed the “Janus Intermediate”, because it represents the point where the enzyme can move forward with productive H2 generation, leading to Nitrogen Fixation or backward with nonproductive H2 generation and relaxation (Fig. 5.2). The name “Janus” refers to the Roman god of transitions with one face looking forward and the other face looking backward.
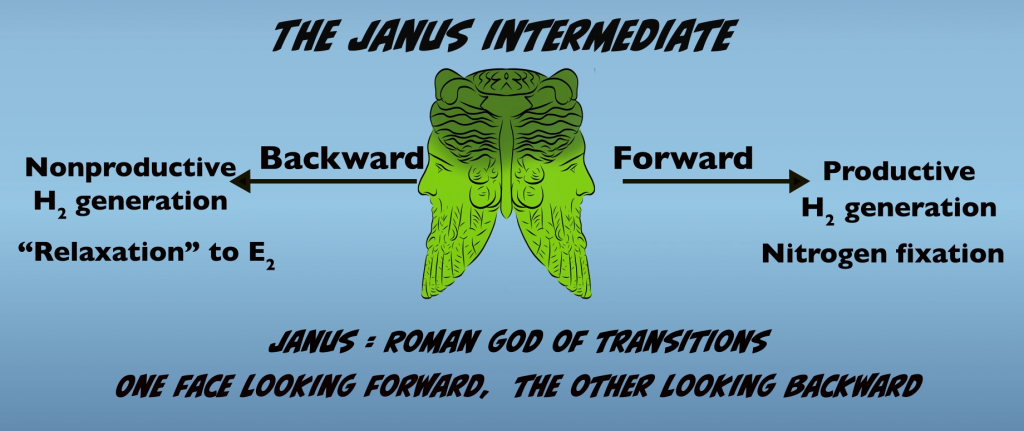
To examine the key processes occurring in the Janus intermediate. We’ll bring our focus down to the atomic level and look at the molecular activity occurring at the iron sulfur reactive face. In the Janus intermediate, the reactive face has accumulated four Hydrogens (Fig. 5.3). Two Hydrogens are in hydride bonds that bridge two iron atoms. These are termed bridging hydrides, and two hydrogens are bound to two sulfur atoms.
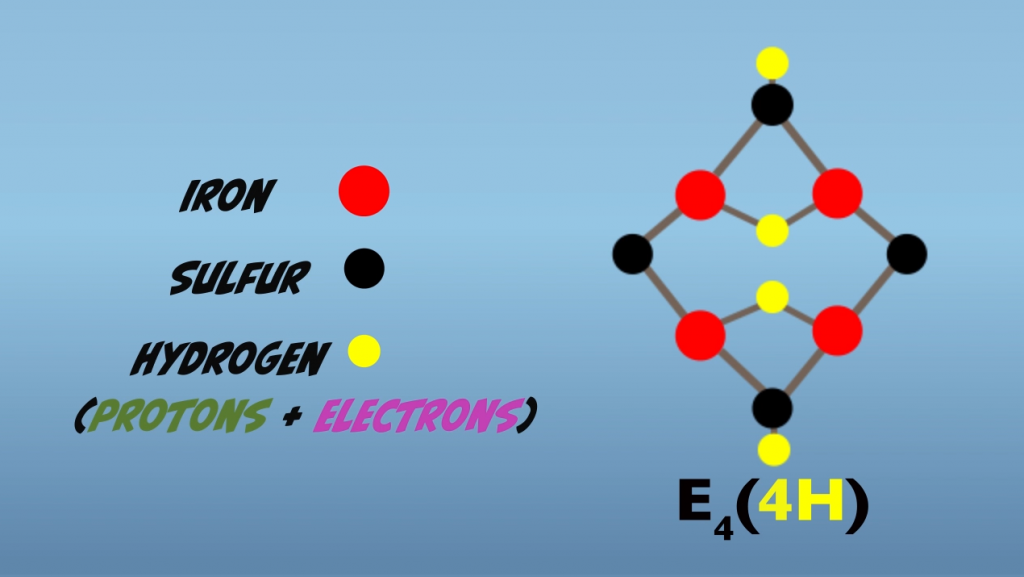
If we look first at the backward reaction, a process called hydride protonation accounts for nonproductive, hydrogen production and relaxation to E2 (Fig. 5.4). This process could also occur with the remaining two hydrogens and result in relaxation from E2 to E0.
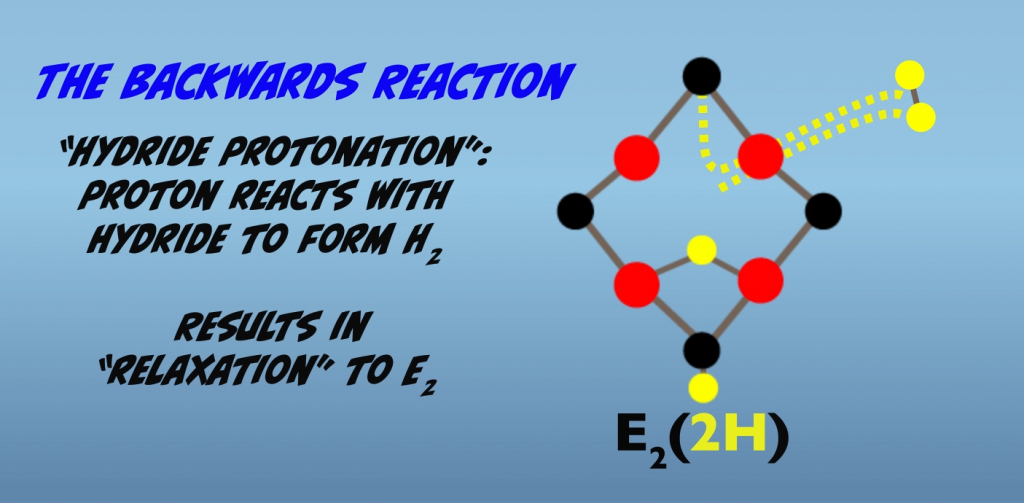
In the forward reaction, three things are occurring in the Janus intermediate: nitrogen binding, breakage of the nitrogen triple bond and the initial reduction of the nitrogen. We’ll take these step-by-step. The presence of N2 is believed to induce the hydrides to form a dihydrogen structure that is bound to a single iron atom (Fig. 5.5).
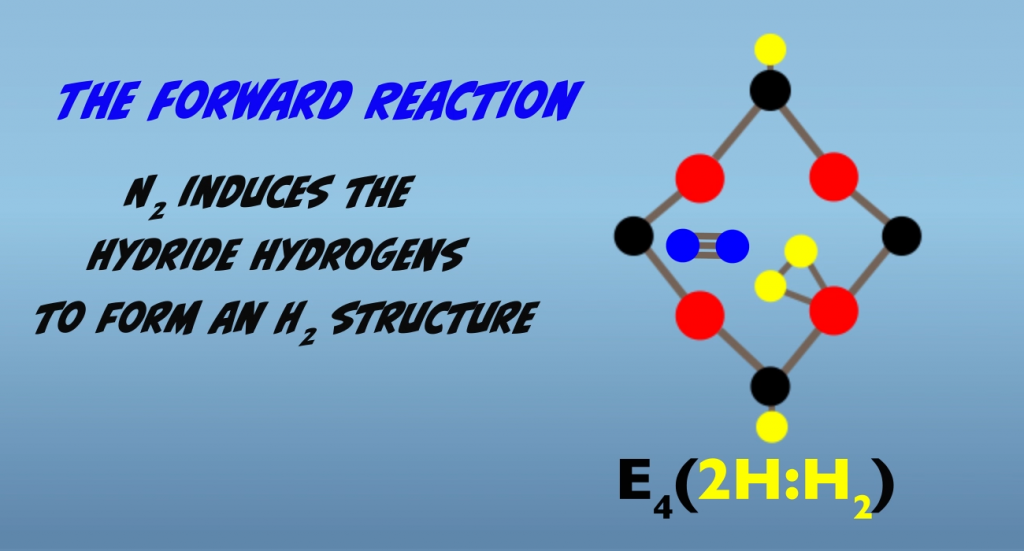
N2 then displaces H2, and H2 departs the cluster in a process termed “reductive elimination” (Fig. 5.6). Loss of H2 by reductive elimination makes the iron atom “super reduced” and activates the cluster for N2 binding.
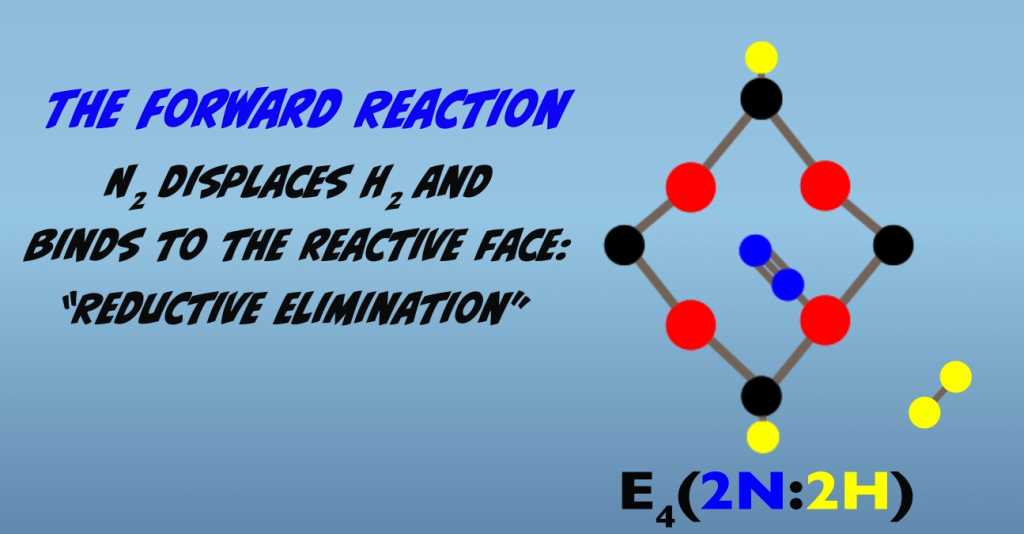
Binding of N2 is then followed immediately by addition of one hydrogen atom to each nitrogen atom (Fig. 5.7). The product has termed a “diazene-level” intermediate.
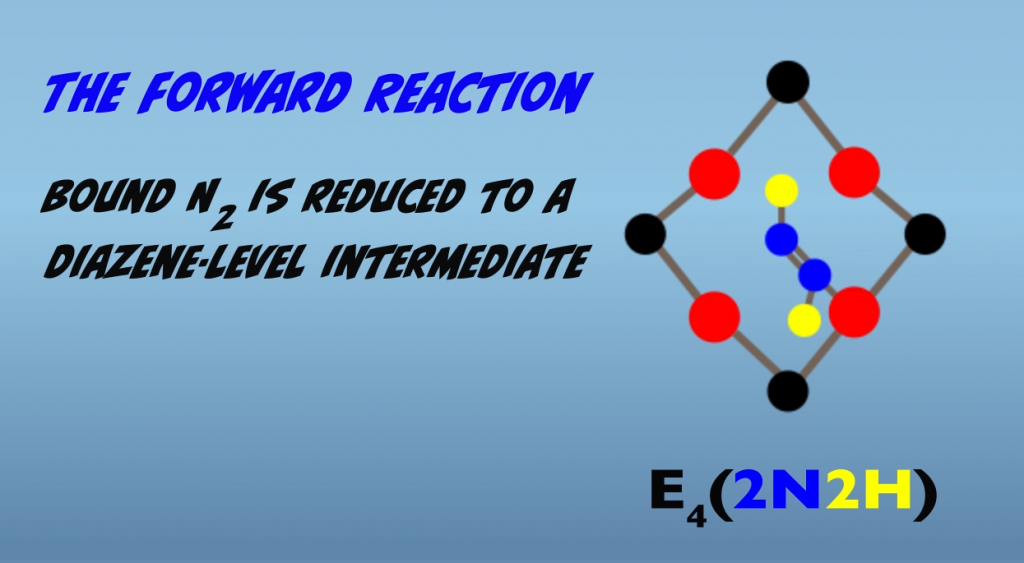
Looking at the energetics of the separate processes helps explain why Nitrogenase employs this mechanism. As illustrated in this graph (Fig. 5.8), N2 bond breakage and reduction to a diazene consumes energy, 67 kilojoules, whereas the formation of H2 releases 170 kilojoules. By coupling these two reactions, the product is a net release of 103 kilojoules, which makes the reaction favorable.
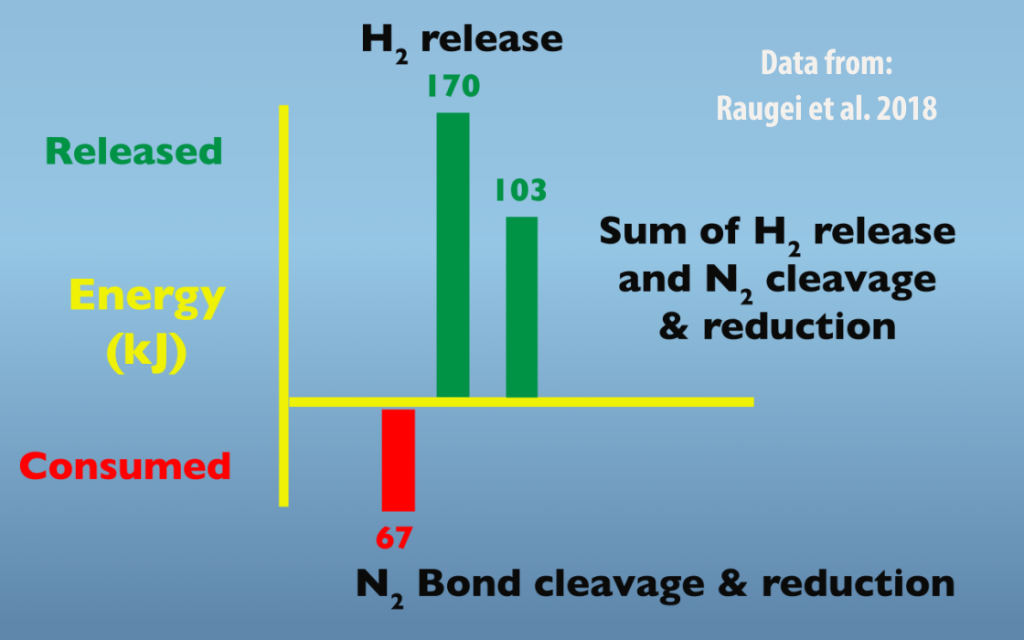
The positive effect of H2 release on the energetics of nitrogen bond breakage and reduction process explains why hydrogen gas formation is an obligate part of Nitrogenase function (Fig. 5.9). It’s important to note that one H2 formed per N2 fixed is a minimum because of the reductive elimination mechanism. Actual amounts of H2 formed relative to N2 fixed will be greater because of relaxation that occurs from E4 down to E2 and potentially from E2 to E0.
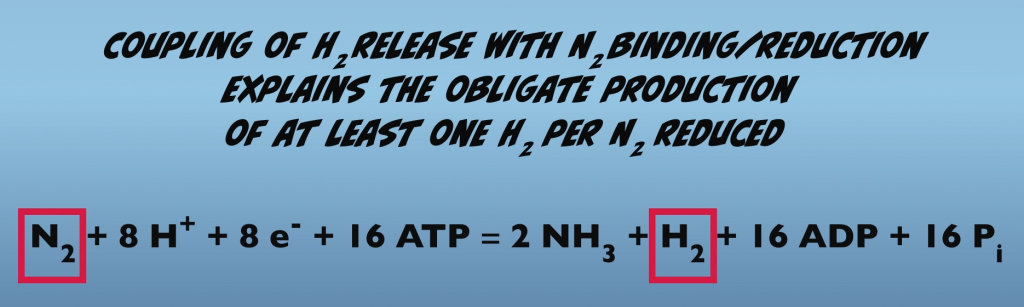
Proceeding further from the Janus intermediate, four additional interactions with the iron protein result in further reduction of the bound nitrogen atoms. As mentioned earlier, the exact nature of the intermediate is not established. So what we’ll look at next are hypothesized intermediates for the proposed alternating pathway. In this pathway, a subsequent electron transfer generates a hydrazido at E5, (Fig. 5.10) and then hydrazine at E6 (Fig. 5.11). At E7, reduction of the terminal nitrogen atom is completed and is released as ammonia, leaving a single bound nitrogen atom as an amide (Fig. 5.12). The eighth electron transfer completes the process releasing the second nitrogen as ammonia (Fig. 5.13). The M-cluster and the molybdenum iron protein are returned to the resting state, E0.
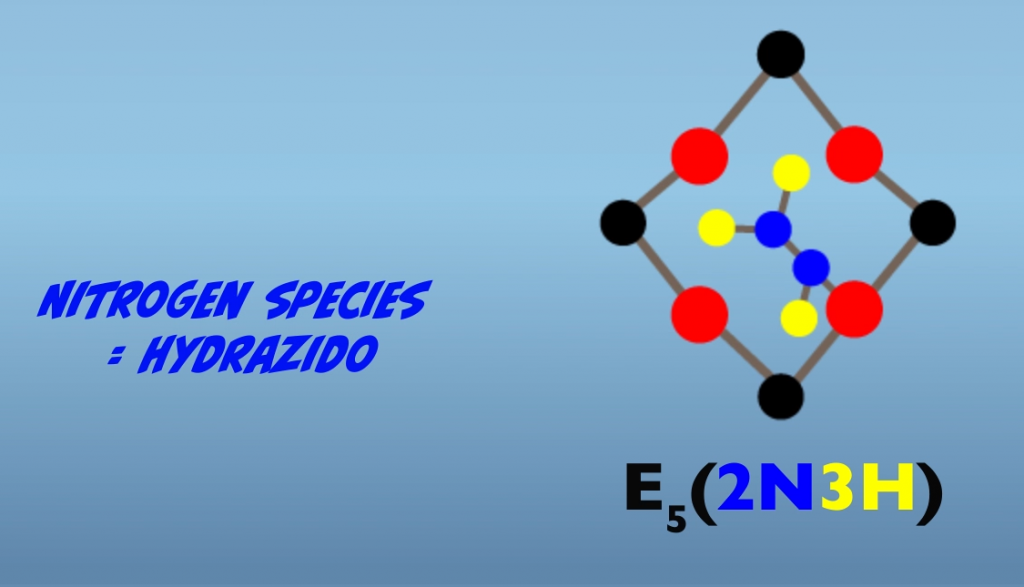
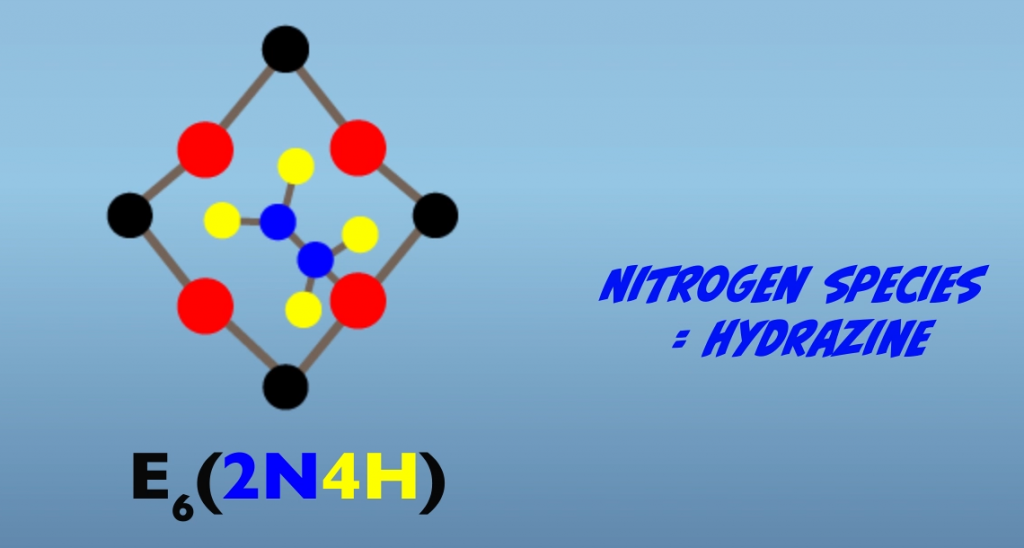
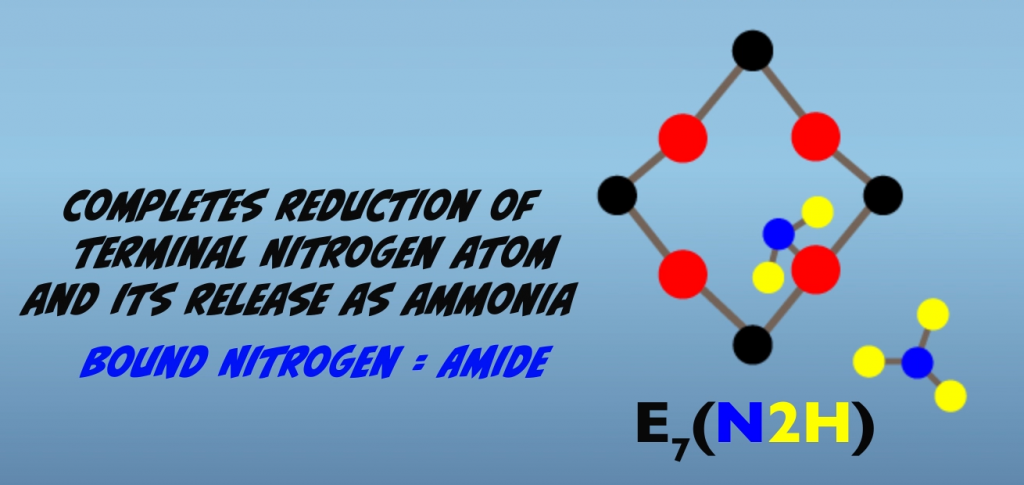
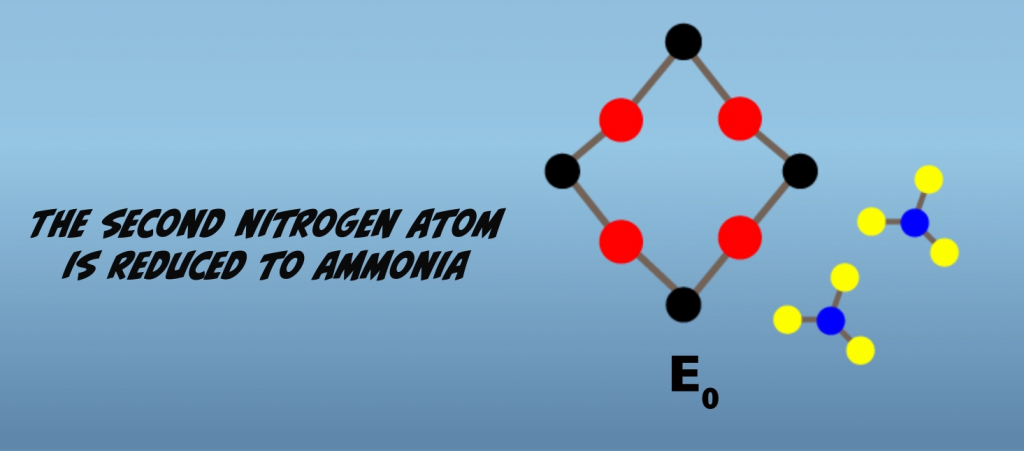