4 Electrophilic Addition of HX to Alkenes
The C=C double bond in alkenes is electron rich: it contains four electrons instead of the two in a single bond. Moreover, the two electrons in the π bonding orbital are higher in energy than those in the σ bonding orbitals, and are positioned above and below the molecular plane. These characteristics make them energetically and physically more accessible for reactions.
Therefore, an alkene can act like a nucleophile during an electrophilic addition reaction, donating a pair of electrons to an electrophile, resulting in the π bond breaking and formation of σ bonds.
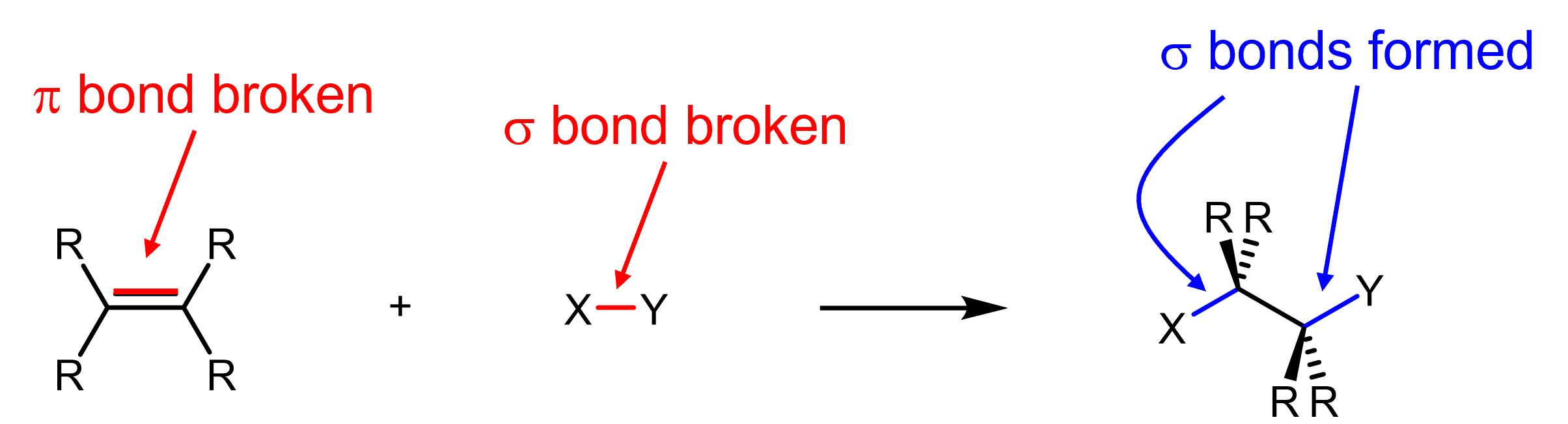
There are many types of electrophilic addition, but for now we will focus on the addition of hydrogen halides (HX) that are strong acids, in other words, HI, HBr, and HCl. Note that many of the basic ideas discussed here will be applicable to other electrophilic addition reactions.
Representing the Reaction
The reaction is generally written in the following manner. (click on the “i” in the image to see additional information)
You will notice some differences between this and how balanced chemical reaction equations are written in general chemistry. Notably, the reagent is on top of the reaction arrow instead of with the organic reactant (also known as substrate). Also, the emphasis here is not about balancing the reaction, but rather to show the chemical transformation from the organic substrate to the organic product.
In this reaction as shown, the H from HBr is added to the left carbon of the original alkene, while Br is added to the right carbon. If you were to draw the product where the H is added to the right carbon and Br to the left carbon, you’d have the exact same product, namely 2-bromo-2,3-dimethylbutane. This is because the example alkene substrate is symmetric; we will discuss the case of asymmetric alkene in the next section.
Reaction Mechanism
The HX addition to alkene reaction mechanism has two steps. The overall reaction energy diagram as shown below.
The reaction is initiated by the overlap of the filled alkene π bonding orbital with the empty HBr σ antibonding orbital (Figure 3). The flow of electron density from the π bonding orbital to the σ antibonding orbital leads to the formation of a high energy transition state (TS1), where a C-H σ bond is in the process of forming while the H-Br σ bond and C-C π bond are breaking (click on “i” above TS1 in figure 2 to see the transition state structure.)

Figure 4 shows how to write the first step of the reaction mechanism with curved arrows: the pair of electrons in the π bond of the alkene go toward the H of HBr, forming a new C-H σ bond between the H from HBr and one of the carbon from the double bond. Concurrently, the pair of electrons from the H-Br σ bond move onto Br.

The species formed from step 1 are a bromide anion and a carbocation. These are reaction intermediates and are located in the local minimum between the two transition states in Figure 2. In the carbocation, one of the two carbons that were part of the alkene double bond is now sp3 hybridized, bonded to two methyl groups and an H. The other carbon is still sp2 hybridized, bonded to only two methyl groups. It is electron deficient with an empty unhybridized p orbital, and we denote this in the line drawing by placing a positive charge on that carbon.
The carbocation and product, making it unstable—it reacts readily and rapidly, never accumulating in any significant concentrations during the reaction.
This step is an Bronsted-Lowry acid-base reaction, with HBr acting as the acid and alkene as the base. Although the alkene is a very weak base, it can be protonated to a small extent by a strong acid. However, the products, bromide and carbocation, are higher in energy relative to the reactants (Figure 2), meaning that the carbocation is more acidic than HBr and the reverse reaction is favored [link to relevant acid/base section]. This is denoted in Figure 4 by an reversible arrow favoring the reactant side [match wording with acid/base section].
The second step of the mechanism involves the overlap of the empty carbocation p orbital with the filled bromide p orbital, Figure 5, forming a new C-Br σ bond. The second transition state geometry (TS2 in Figure 2) simply involves the formation of this bond.
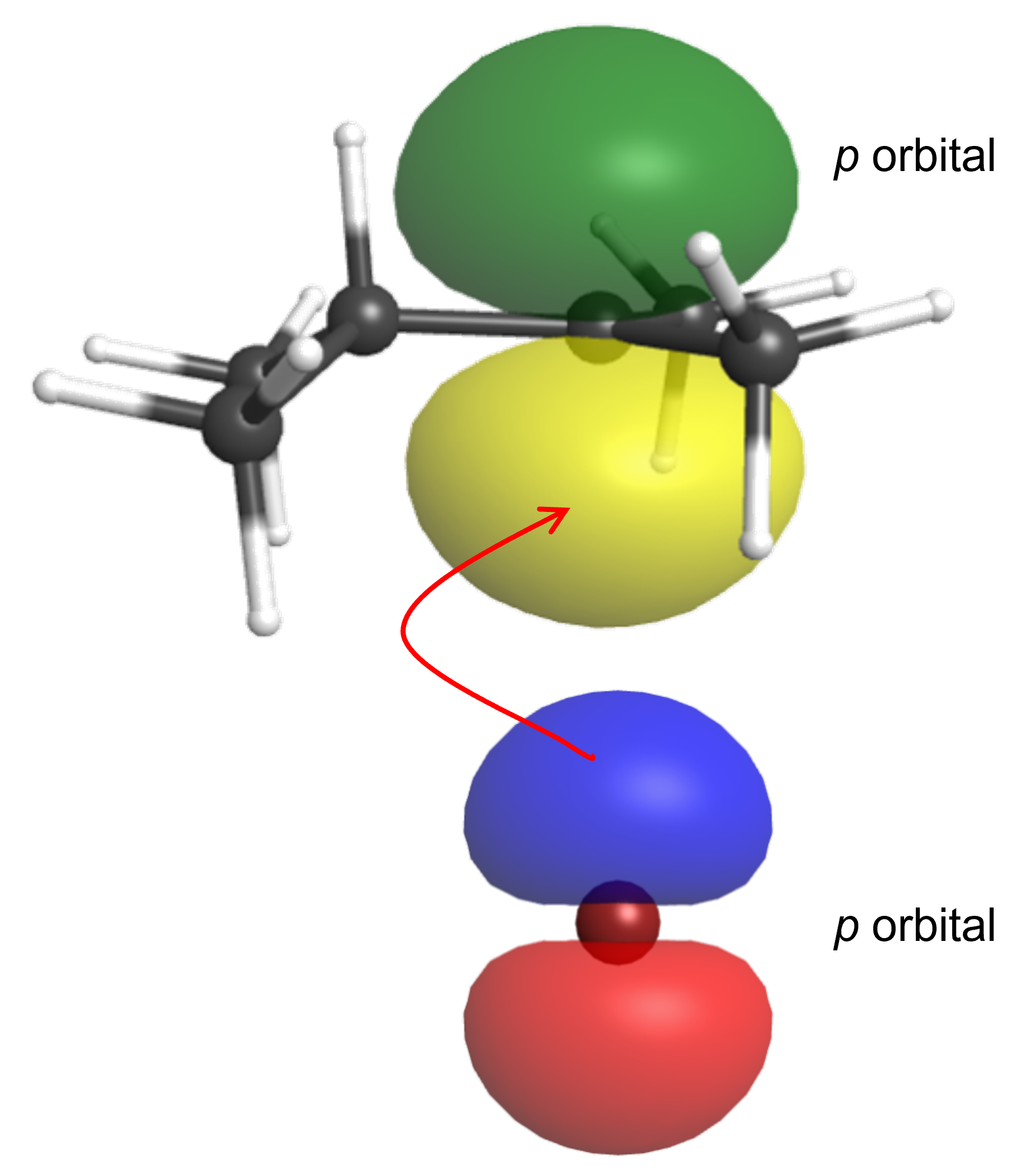
Figure 6 shows how to write the second step of the reaction mechanism with curved arrows: the electrophilic carbocation accepts a pair of electrons from the nucleophilic Br– anion, forming the neutral alkyl halide product. Note that all the carbons in the product molecule are sp3 hybridized.
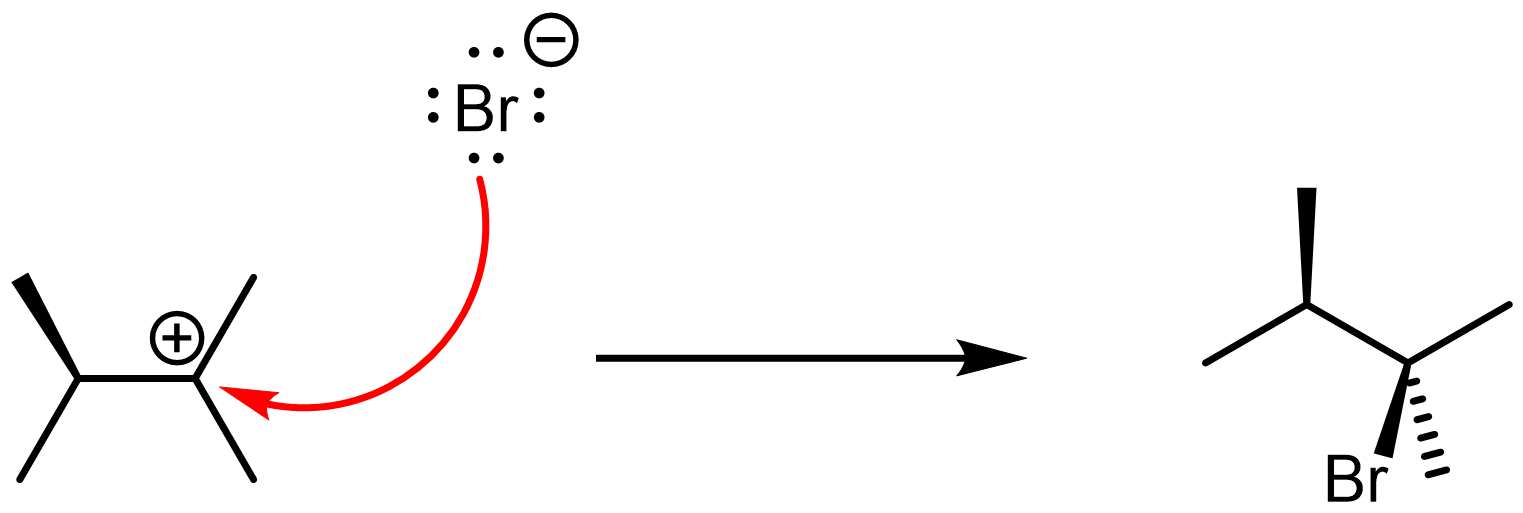
Step 2 is a Lewis acid-base reaction, with the carbocation acting as a very strong Lewis acid (its empty p orbital can easily accept electron pairs during reactions). It occurs readily, and is essentially forward-direction only.
As Figure 2 shows, the activation energy of step 1 (Ea,1) is much larger than the activation energy of step 2 (Ea,2), making step 1 the slow step in the reaction mechanism. Hence, the rate of the overall reaction is limited by step 1, making it the rate-determining step (RDS). Understanding the energetics of species involved in this step will be crucial for understanding the overall reaction, and we will explore these concepts in detail in the following sections.