49 Molecule Polarity (M9Q2)
Introduction
This section explores how to determine a molecule’s polarity, as well as how polarity can give us insight into a molecule’s properties. We will first define molecular polarity and how it can be observed in a molecule’s bonds, as well as its overall properties. The section below provides a more detailed description of these topics, worked examples, practice problems, and a glossary of important terms.
Learning Objectives for Molecule Polarity
- Predict molecular polarity and molecular dipole moments by considering molecular shape and bond polarity.
| Molecule Polarity and Dipole Moment | Properties of Polar Molecules |
| Key Concepts and Summary | Glossary | End of Section Exercises |
Molecular Polarity and Dipole Moment
As discussed previously, polar covalent bonds connect two atoms with differing electronegativities, leaving one atom with a partial positive charge (δ+) and the other atom with a partial negative charge (δ–), as the electrons are pulled toward the more electronegative atom. This separation of charge gives rise to a bond dipole moment. The magnitude of a bond dipole moment is represented by the Greek letter mu (µ) and is given by the formula shown here, where Q is the magnitude of the partial charges (determined by the electronegativity difference) and r is the distance between the charges:
μ = Qr
This bond moment can be represented as a vector, a quantity having both direction and magnitude (Figure 1). Dipole vectors are shown as arrows pointing along the bond from the less electronegative atom toward the more electronegative atom. A small plus sign is drawn on the less electronegative end to indicate the partially positive end of the bond. The length of the arrow is proportional to the magnitude of the electronegativity difference between the two atoms.
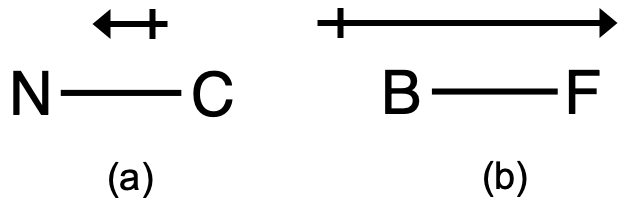
A whole molecule may also have a separation of charge, depending on its molecular structure and the polarity of each of its bonds. If such a charge separation exists, the molecule is said to be a polar molecule; otherwise the molecule is said to be nonpolar. The dipole moment measures the extent of net charge separation in the molecule as a whole. We determine the dipole moment by adding the bond moments in three-dimensional space, taking into account the molecular structure.
For diatomic molecules, there is only one bond, so its bond dipole moment determines the molecular polarity. Homonuclear diatomic molecules such as Br2 and N2 have no difference in electronegativity, so their dipole moment is zero. For heteronuclear molecules such as CO, there is a small dipole moment. For HF, there is a larger dipole moment because there is a larger difference in electronegativity. Recall that we also discussed electronegativity differences leading to polar covalent bonds in the previous module, so this should be a relatively familiar topic.
When a molecule contains more than one bond, the geometry must be taken into account. If the bonds in a molecule are arranged such that their bond moments cancel (vector sum equals zero), then the molecule is nonpolar. This is the situation in CO2 (Figure 2, left). Each of the bonds is polar, but the molecule as a whole is nonpolar. From the Lewis structure, and using VSEPR theory, we determine that the CO2 molecule is linear with polar C=O bonds on opposite sides of the carbon atom. The bond moments cancel because they are pointed in opposite directions. In the case of the water molecule (Figure 2, right), the Lewis structure again shows that there are two bonds to a central atom, and the electronegativity difference again shows that each of these bonds has a nonzero bond moment. In this case, however, the molecular structure is bent because of the lone pairs on O, and the two bond moments do not cancel. Therefore, water does have a net dipole moment and is a polar molecule (has a dipole).
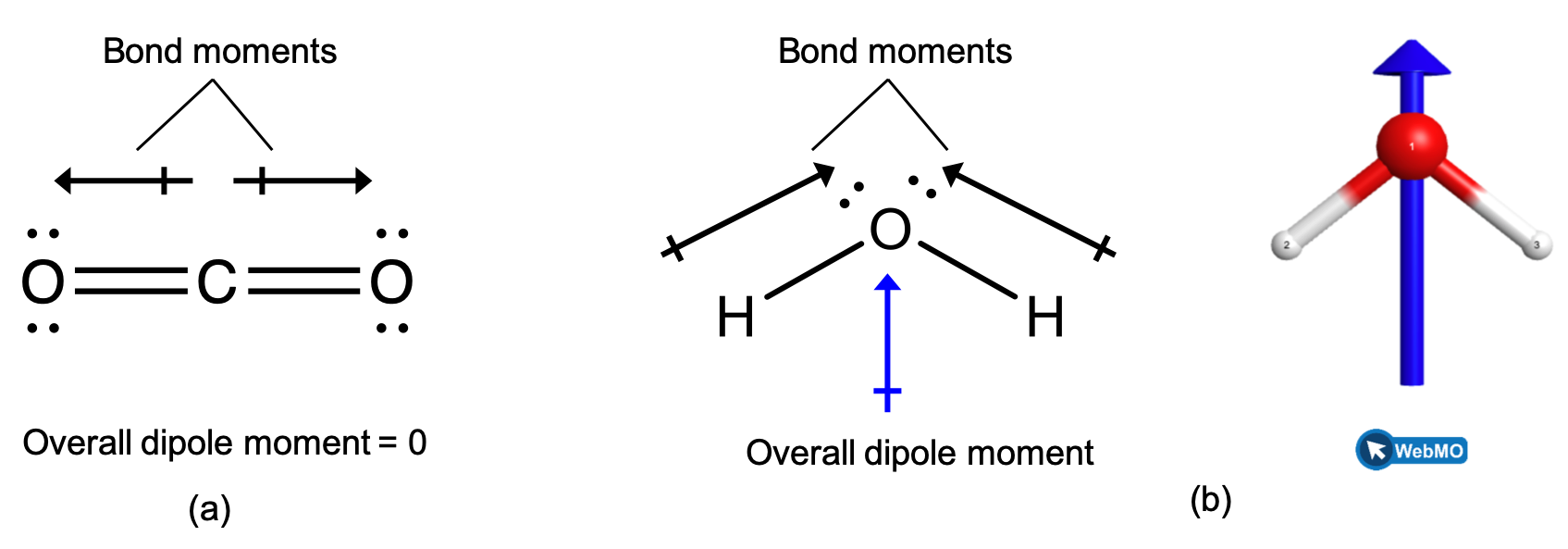
The OCS molecule has a structure similar to CO2, but a sulfur atom has replaced one of the oxygen atoms. To determine if this molecule is polar, we draw the molecular structure. VSEPR theory predicts a linear molecule:
The C-O bond is considerably polar. Although C and S have very similar electronegativity values, S is slightly more electronegative than C, and so the C-S bond is just slightly polar. Because oxygen is more electronegative than sulfur, the oxygen end of the molecule is the negative end.
Chloromethane, CH3Cl, is another example of a polar molecule. Although the polar C–Cl and C–H bonds are arranged in a tetrahedral geometry, the C–Cl bond has a larger bond moment than the C–H bonds, and the bond moments do not cancel each other. All of the dipoles have an upward component in the orientation shown, since carbon is more electronegative than hydrogen and less electronegative than chlorine. The horizontal components of the C-H bond moments would cancel each other out and the overall dipole moment would therefore be pointing upward in the molecule as shown.
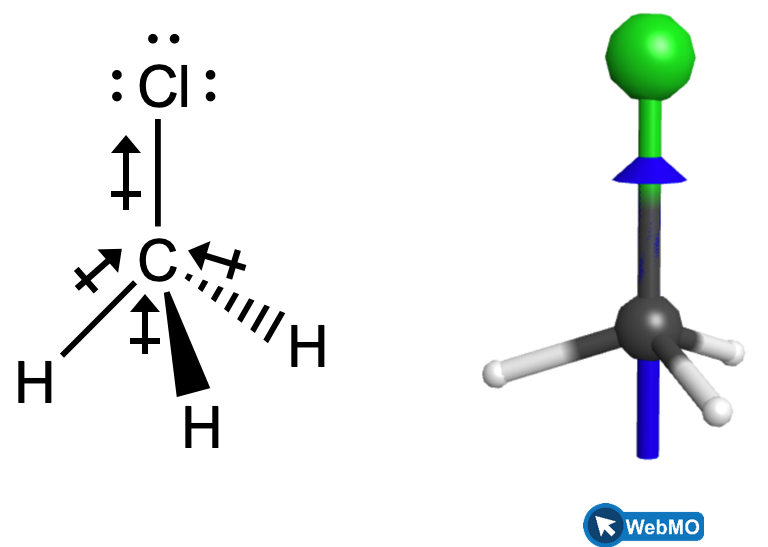
When we examine the highly symmetrical molecules BH3 (trigonal planar), CH4 (tetrahedral), PF5 (trigonal bipyramidal), and SF6 (octahedral), in which all the polar bonds are identical, the molecules are nonpolar. The bonds in these molecules are arranged such that their dipoles cancel. However, just because a molecule contains identical bonds does not mean that the dipoles will always cancel. Many molecules that have identical bonds and lone pairs on the central atoms have bond dipoles that do not cancel. Examples include H2S (Figure 4) and NH3 (Figure 5). A hydrogen atom is at the positive end and a nitrogen or sulfur atom is at the negative end of the polar bonds in these molecules:
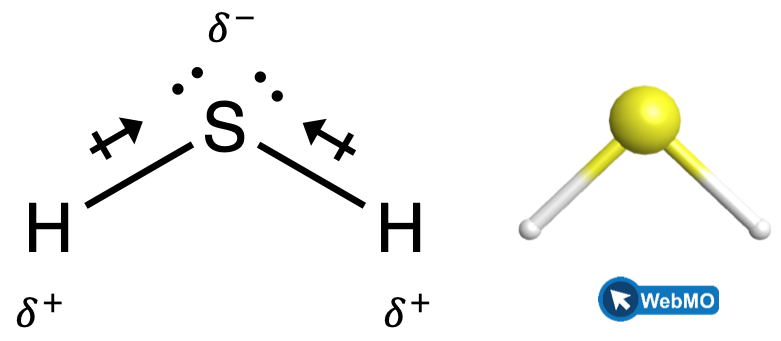
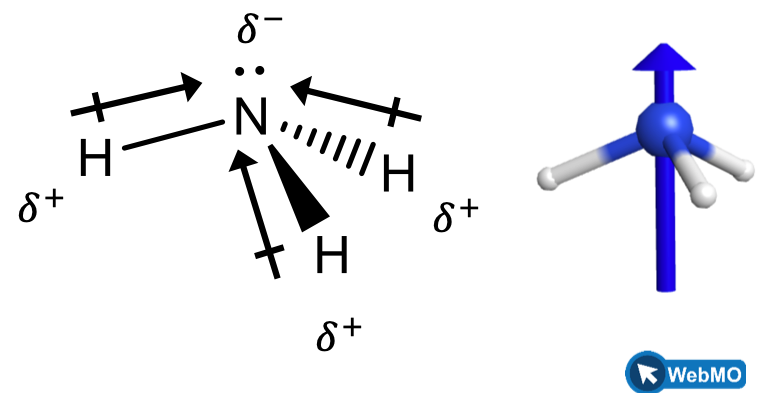
To summarize, to be polar, a molecule must:
- Contain at least one polar covalent bond.
- Have a molecular structure such that the sum of the vectors of each bond dipole moment does not cancel.
Properties of Polar Molecules
Polar molecules tend to align when placed in an electric field with the positive end of the molecule oriented toward the negative plate and the negative end toward the positive plate (Figure 6). We can use an electrically charged object to attract polar molecules, but nonpolar molecules are not attracted. Also, polar solvents are better at dissolving polar substances, and nonpolar solvents are better at dissolving nonpolar substances.
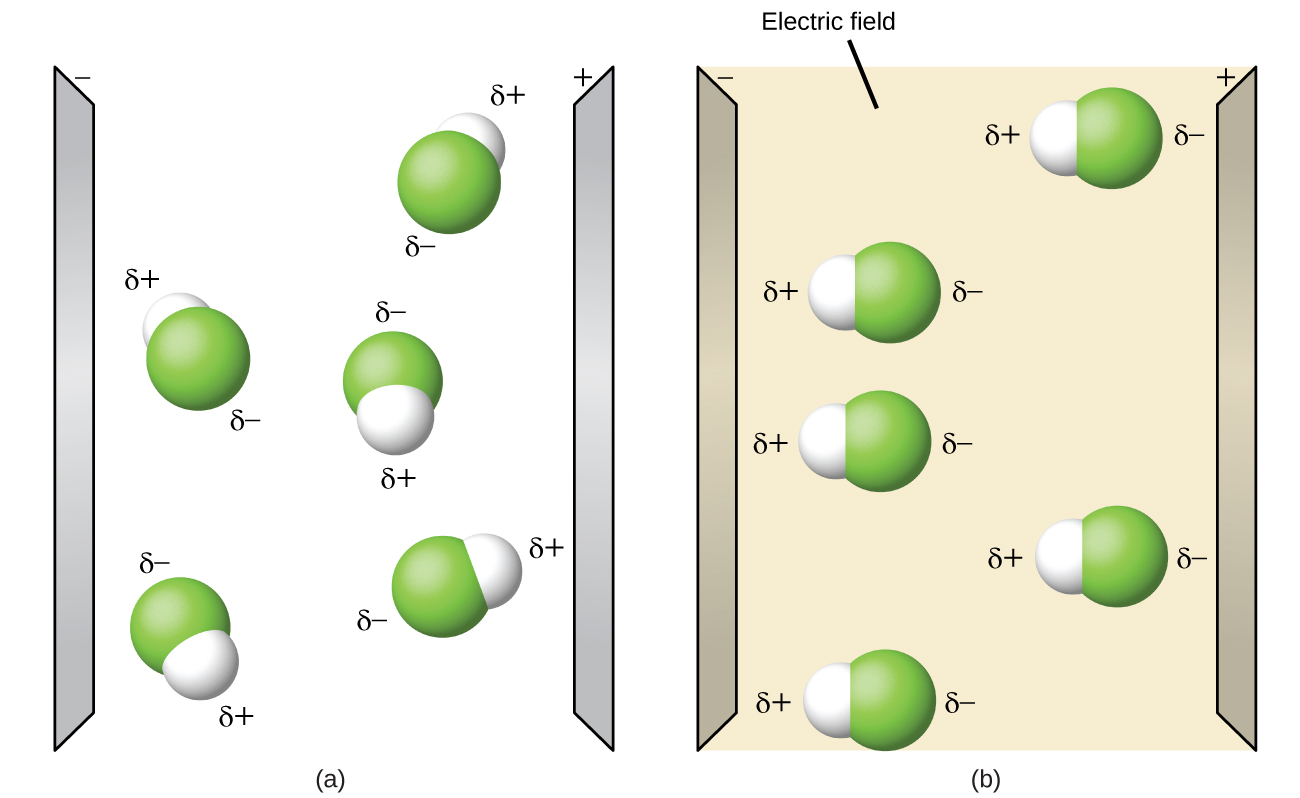
Demonstration: Polar molecules heat up in a microwave
Set up. In the following demonstration, there are samples of solid H2O and solid CO2. As shown in Figure 2 above, H2O is a polar molecule and CO2 is a nonpolar molecule. As stated above, when polar molecules are exposed to an electric field, they attempt to orient themselves within the electric field. A transmitter in a microwave oven produces an electromagnetic field in the microwave region of the electromagnetic spectrum. Recall from an earlier module that electromagnetic radiation is composed of perpendicular oscillating electric and magnetic fields. The oscillating electric field in a typical microwave reverses polarity approximately 2.45 billion times per second (2.45 GHz). With each oscillation, polar molecules attempt to align with the electric field. Switching direction causes the polar molecules to twist back and forth, gaining energy and heating up.
Explanation. As seen in this demonstration, the polar sample (solid H2O) heated up in the microwave and melted into liquid H2O. The nonpolar sample (solid CO2) did not change phase when put into a microwave oven since the oscillating electric field did not have an effect on the nonpolar molecules.
The molecule polarity simulation provides many ways to explore dipole moments of bonds and molecules.
Example 1
Polarity Simulations
Open the molecule polarity simulation and select the “Three Atoms” tab at the top. This should display a molecule ABC with three electronegativity adjustors. You can display or hide the bond moments, molecular dipoles, and partial charges at the right. Turning on the electric field will show whether the molecule moves when exposed to a field, similar to Figure 6.
Use the electronegativity controls to determine how the molecular dipole will look for the starting bent molecule if:
(a) A and C are very electronegative and B is in the middle of the range.
(b) A is very electronegative, and B and C are not.
Solution
(a) Molecular dipole moment points immediately between A and C.
(b) Molecular dipole moment points along the A–B bond, toward A.
Check Your Learning
Determine the partial charges that will give the largest possible bond dipoles.
Answer:
The largest bond moments will occur with the largest partial charges. The two solutions above represent how unevenly the electrons are shared in the bond. The bond moments will be maximized when the electronegativity difference is greatest. The controls for A and C should be set to one extreme, and B should be set to the opposite extreme. Although the magnitude of the bond moment will not change based on whether B is the most electronegative or the least, the direction of the bond moment will.
Key Concepts and Summary
A dipole moment measures a separation of charge. For one bond, the bond dipole moment is determined by the difference in electronegativity between the two atoms. For a molecule, the overall dipole moment is determined by both the individual bond moments and how these dipoles are arranged in the molecular structure. Polar molecules (those with an appreciable dipole moment) interact with electric fields, whereas nonpolar molecules do not.
Glossary
- bond dipole moment
- separation of charge in a bond that depends on the difference in electronegativity and the bond distance represented by partial charges or a vector
- dipole moment
- property of a molecule that describes the separation of charge determined by the sum of the individual bond moments based on the molecular structure
- polar molecule
- (also, dipole) molecule with an overall dipole moment
-
- vector
- quantity having magnitude and direction
Chemistry End of Section Exercises
- Explain how a molecule that contains polar bonds can be nonpolar.
- Determine the electron-pair geometry and molecular geometry for each of the following molecules and ions. Which of these molecules and ions contain polar bonds? Which of these molecules and ions have dipole moments?
- H3O+
- PCl4−
- SnCl3−
- BrCl4−
- ICl3
- XeF4
- SF2
- Determine the electron-pair geometry and molecular geometry for each of the following molecules. Identify the molecules with a dipole moment:
- SF4
- CF4
- Cl2CCBr2
- CH3Cl
- H2CO
- Determine the electron-pair geometry and molecular geometry for each of the following molecules. Which of the following molecules have dipole moments?
- CS2
- SeS2
- CCl2F2 (C is the central atom)
- PCl3 (P is the central atom)
- ClNO (N is the central atom)
- The molecule XF3 has a dipole moment. Is X boron or phosphorus?
- There are three possible structures for PCl2F3 with phosphorus as the central atom. Draw them and discuss how measurements of dipole moments could help distinguish among them.
- Is the Cl2BBCl2 molecule polar or nonpolar?
- Use the simulation to perform the following exercises for a two-atom molecule:
- Adjust the electronegativity value so the bond dipole is pointing toward B. Then determine what the electronegativity values must be to switch the dipole so that it points toward A.
- With a partial positive charge on A, turn on the electric field and describe what happens.
- With a small partial negative charge on A, turn on the electric field and describe what happens.
- Reset all, and then with a large partial negative charge on A, turn on the electric field and describe what happens.
- Use the simulation to perform the following exercises for a real molecule. You may need to rotate the molecules in three dimensions to see certain dipoles.
- Sketch the bond dipoles and molecular dipole (if any) for O3. Explain your observations.
- Look at the bond dipoles for NH3. Use these dipoles to predict whether N or H is more electronegative.
- Predict whether there should be a molecular dipole for NH3 and, if so, in which direction it will point. Check the molecular dipole box to test your hypothesis.
Answers to Chemistry End of Section Exercises
- As long as the polar bonds are compensated (for example, two identical atoms are found directly across the central atom from one another), the molecule can be nonpolar.
- (a) H3O+: electron-pair geometry (EPG) = tetrahedral, molecular geometry (MG) = trigonal pyramidal, polar bonds, dipole moment
(b) PCl4–: EPG = trigonal bipyramidal, MG = see-saw, polar bonds, dipole moment
(c) SnCl3–: EPG = tetrahedral, MG = trigonal pyramidal, polar bonds, dipole moment
(d) BrCl4–: EPG = octahedral, MG = square planar, nonpolar bonds, no dipole moment
(e) ICl3: EPG = trigonal bipyramidal, MG = T-shaped, polar bonds, dipole moment
(f) XeF4: EPG = octahedral, MG = square planar, polar bonds, no dipole moment
(g) SF2: EPG = tetrahedral, MG = bent, polar bonds, dipole moment - (a) SF4: EPG = trigonal bipyramidal, MG = see-saw, polar bonds, dipole moment
(b) CF4: EPG = tetrahedral, MG = tetrahedral, polar bonds, no dipole moment
(c) Cl2CCBr2: EPG (either C as central atom) = trigonal planar, MG (either C as central atom) = trigonal planar, polar bonds, dipole moment (C-Cl dipoles are stronger than the C-Br dipoles, so they will not cancel)
(d) CH3Cl: EPG = tetrahedral, MG = tetrahedral, polar bonds, dipole moment (C-Cl bond is more polar than C-H bonds)
(e) H2CO: EPG = trigonal planar, MG = trigonal planar, polar bonds, dipole moment (C-O bond is more polar than C-H bonds) - (a) CS2: EPG = linear, MG = linear, nonpolar bonds, no dipole moment
(b)SeS2: EPG = trigonal planar, MG = bent, nonpolar bonds, no dipole moment
(c) CCl2F2: EPG = tetrahedral, MG = tetrahedral, polar bonds, dipole moment (C-F bonds are more polar than C-Cl bonds)
(d) PCl3: EPG = tetrahedral, MG = trigonal pyramidal, polar bonds, dipole moment
(e) ClNO: EPG = trigonal planar, MG = bent, polar bonds, dipole moment - X must be phosphorus. If X were boron, even though the B-F bonds would be polar, the molecular geometry of BF3 would be trigonal planar, which would not produce a polar molecule. If X is P, PF3 would have a trigonal pyramidal molecular geometry and polar bonds, leading to a dipole moment.
- The three possible arrangements are shown below and differ in whether the Cl, F, or one of each is placed in the axial positions of the trigonal bipyramidal geometry. Measuring the dipole moments of these structure, structure (1) would not have any dipole moment since the P-Cl polar bonds would cancel, and the P-F polar bonds would also cancel. Structure (2) would have the largest dipole moment because none of the P-F polar bonds exactly cancel with P-F polar bonds. The P-Cl polar bonds do cancel some of the P-F bond polarity. Structure (3) has an intermediate dipole moment since the two axial F atoms directly cancel and then it is the difference between a single F atom vs 2 Cl atoms in the equatorial positions.
- nonpolar
- With a partial negative charge on A and the electric field turned on, the molecule rotates to have the partial negative charge facing toward the positively charged plate in the electric field. Clearing everything and starting from the beginning, then putting a large partial negative charge on A causes the molecule to rotate faster.
- The bond dipoles for NH3 indicate that N is more electronegative. The molecular dipole points away from the hydrogen atoms.