55 Vapor Pressure and Boiling Point Correlations (M10Q3)
Introduction
Learning Objectives for Vapor Pressure Comparisons
- Connect trends in physical properties with relative strength of intermolecular forces.
- Relate vapor pressure and normal boiling point to strength of intermolecular forces.
| Vapor Pressure and IMFs | Boiling Point and IMFs |
- Relate vapor pressure and normal boiling point to strength of intermolecular forces.
| Key Concepts and Summary | Glossary | End of Section Exercises |
Vapor Pressure and IMFs
When a liquid vaporizes in a closed container, gas molecules cannot escape. As these gas phase molecules move randomly about, they will occasionally collide with the surface of the condensed phase, and in some cases, these collisions will result in the molecules re-entering the condensed phase. The change from the gas phase to the liquid is called condensation. When the rate of condensation becomes equal to the rate of vaporization, neither the amount of the liquid nor the amount of the vapor in the container changes. The vapor in the container is then said to be in equilibrium with the liquid. Keep in mind that this is not a static situation, as molecules are continually exchanged between the condensed and gaseous phases. Such is an example of a dynamic equilibrium, the status of a system in which opposite processes (for example, vaporization and condensation) occur at equal rates. The pressure exerted by the vapor in equilibrium with a liquid in a closed container at a given temperature is called the liquid’s vapor pressure (or equilibrium vapor pressure). The area of the surface of the liquid in contact with a vapor and the size of the vessel have no effect on the vapor pressure, although they do affect the time required for the equilibrium to be reached. We can measure the vapor pressure of a liquid by placing a sample in a closed container, like that illustrated in Figure 1, and using a manometer to measure the increase in pressure that is due to the vapor in equilibrium with the condensed phase.
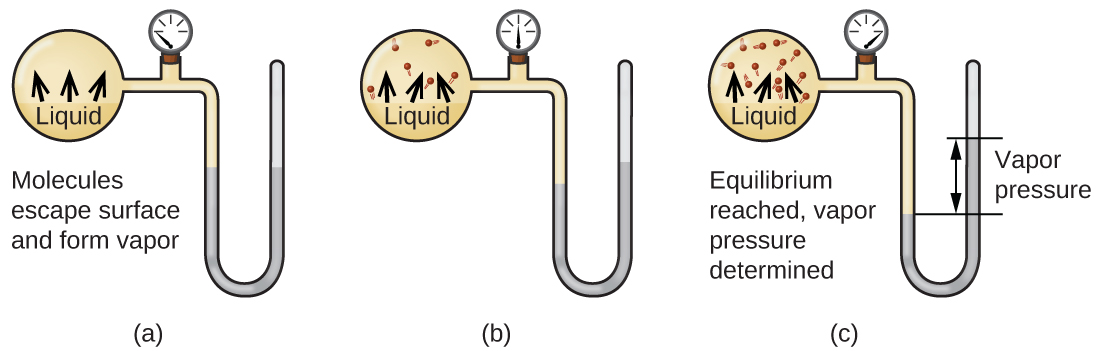
The chemical identities of the molecules in a liquid determine the types (and strengths) of intermolecular attractions possible; consequently, different substances will exhibit different equilibrium vapor pressures. Relatively strong intermolecular attractive forces will serve to impede vaporization as well as favoring “recapture” of gas-phase molecules when they collide with the liquid surface, resulting in a relatively low vapor pressure. Weak intermolecular attractions present less of a barrier to vaporization, and a reduced likelihood of gas recapture, yielding relatively high vapor pressures. The following example illustrates this dependence of vapor pressure on intermolecular attractive forces.
Example 1
Explaining Vapor Pressure in Terms of IMFs
Given the shown structural formulas for these four compounds, explain their relative vapor pressures in terms of types and extents of IMFs:
Solution
Diethyl ether has a very small dipole and most of its intermolecular attractions are London forces. Although this molecule is the largest of the four under consideration, its IMFs are the weakest and, as a result, its molecules most readily escape from the liquid. It also has the highest vapor pressure. Due to its smaller size, ethanol exhibits weaker dispersion forces than diethyl ether. However, ethanol is capable of hydrogen bonding and, therefore, exhibits stronger overall IMFs, which means that fewer molecules escape from the liquid at any given temperature, and so ethanol has a lower vapor pressure than diethyl ether. Water is much smaller than either of the previous substances and exhibits weaker dispersion forces, but its extensive hydrogen bonding provides stronger intermolecular attractions, fewer molecules escaping the liquid, and a lower vapor pressure than for either diethyl ether or ethanol. Ethylene glycol has two −OH groups, so, like water, it exhibits extensive hydrogen bonding. It is much larger than water and thus experiences larger London forces. Its overall IMFs are the largest of these four substances, which means its vaporization rate will be the slowest and, consequently, its vapor pressure will be the lowest.
Check Your Learning
At 20 °C, the vapor pressures of several alcohols are given in this table. Explain these vapor pressures in terms of types and extents of IMFs for these alcohols:
Compound | methanol CH3OH | ethanol C2H5OH | propanol C3H7OH | butanol C4H9OH |
Vapor Pressure at 20 °C | 11.9 kPa | 5.95 kPa | 2.67 kPa | 0.56 kPa |
Table 1. |
Answer:
All these compounds exhibit hydrogen bonding; these strong IMFs are difficult for the molecules to overcome, so the vapor pressures are relatively low. As the size of molecule increases from methanol to butanol, dispersion forces increase, which means that the vapor pressures decrease as observed:
Pmethanol > Pethanol > Ppropanol > Pbutanol.
As temperature increases, the vapor pressure of a liquid also increases due to the increased average KE of its molecules. Recall that at any given temperature, the molecules of a substance experience a range of kinetic energies, with a certain fraction of molecules having a sufficient energy to overcome IMF and escape the liquid (vaporize). At a higher temperature, a greater fraction of molecules have enough energy to escape from the liquid, as shown in Figure 2. The escape of more molecules per unit of time and the greater average speed of the molecules that escape both contribute to the higher vapor pressure.
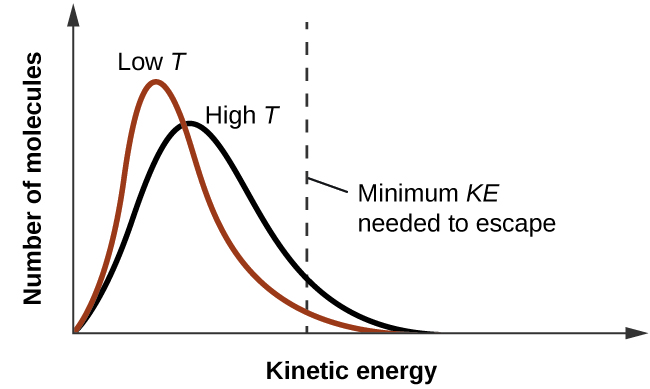
Boiling Point and IMFs
When the vapor pressure increases enough to equal the external atmospheric pressure, the liquid reaches its boiling point. The boiling point of a liquid is the temperature at which its equilibrium vapor pressure is equal to the pressure exerted on the liquid by its gaseous surroundings. For liquids in open containers, this pressure is that due to the earth’s atmosphere. The normal boiling point of a liquid is defined as its boiling point when surrounding pressure is equal to 1 atm (101.3 kPa). When a substance boils, the bubbles that form are filled with the gaseous phase of that substance. A pot of boiling water, therefore, produces bubbles that contain gaseous water. Figure 3 shows the variation in vapor pressure with temperature for several different substances. Considering the definition of boiling point, these curves may be seen as depicting the dependence of a liquid’s boiling point on surrounding pressure.
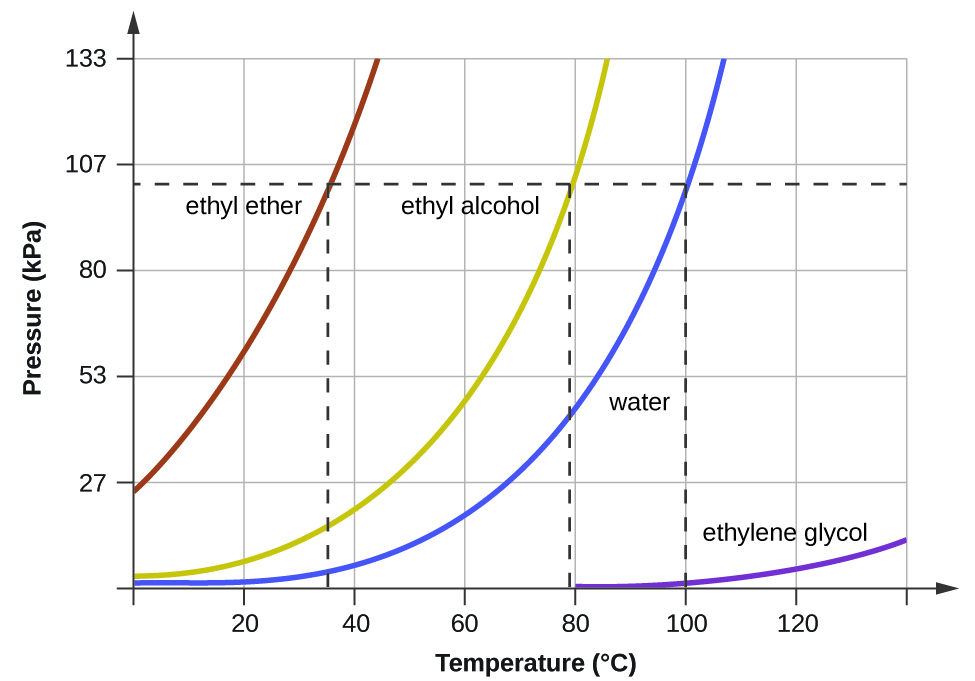
Demonstration: Boiling point and pressure
Set up. As explained above, a liquid boils when its vapor pressure is equal to the pressure of the gaseous surroundings. The following demonstration will illustrate this. On a hot plate, an Erlenmeyer flask filled with water is stoppered and a deflated balloon is attached to the top. The pressure of the external gaseous surroundings in this case is the atmospheric pressure at the time the video was filmed. As the water is heated, the gaseous water vapor will cause the vapor pressure to increase and the gaseous water vapor will fill the balloon.
Prediction. Before watching the video, make a prediction about whether the balloon will inflate slowly as the water is heated to its boiling point, or whether the balloon will inflate only when the boiling point is reached.
Explanation. In this video, the balloon starts to inflate only after the water begins to boil. Until that point, the balloon appears deflated and you’ll notice that for the first 5 minutes, there’s no change in the balloon’s volume. Before the boiling point is reached, the vapor pressure inside the balloon is less than the atmospheric pressure and therefore the balloon cannot be inflated. Once the vapor pressure inside the balloon matches the external vapor pressure (i.e., once the boiling point is reached), the balloon is inflated. As the water begins boiling more rapidly, the balloon inflates more quickly. Remember that the vapor inside the balloon is gaseous water (i.e., H2O(g)) and it is not hot air or gaseous hydrogen (H2(g)) and gaseous oxygen (O2(g)).
Example 2
A Boiling Point at Reduced Pressure
A typical atmospheric pressure in Leadville, Colorado (elevation 10,200 feet) is 68 kPa. Use the graph in Figure 3 to determine the boiling point of water at this elevation.
Solution
The graph of the vapor pressure of water versus temperature in Figure 3 indicates that the vapor pressure of water is 68 kPa at about 90 °C. Thus, at about 90 °C, the vapor pressure of water will equal the atmospheric pressure in Leadville, and water will boil.
Check Your Learning
The boiling point of ethyl ether was measured to be 10 °C at a base camp on the slopes of Mount Everest. Use Figure 3 to determine the approximate atmospheric pressure at the camp.
Answer:
Approximately 40 kPa (0.4 atm)
Key Concepts and Summary
The temperatures at which phase transitions occur are determined by the relative strengths of intermolecular attractions and are, therefore, dependent on the chemical identity of the substance. As temperature of a liquid increases, the vapor pressure of the liquid also increases, since more molecules are able to escape from the liquid phase and enter the gas phase. A substance boils when the vapor pressure of the liquid exceeds the surrounding pressure. The normal boiling point, therefore, is the boiling point at 1 atm of pressure. When a substance boils, the bubbles are filled with the gaseous form of that substance — no chemical reaction has taken place during the phase change.
Glossary
- boiling point
- temperature at which the vapor pressure of a liquid equals the pressure of the gas above it
- condensation
- change from a gaseous to a liquid state
- dynamic equilibrium
- state of a system in which reciprocal processes are occurring at equal rates
- normal boiling point
- temperature at which a liquid’s vapor pressure equals 1 atm (760 torr)
- vapor pressure
- (also, equilibrium vapor pressure) pressure exerted by a vapor in equilibrium with a solid or a liquid at a given temperature
- vaporization
- change from liquid state to gaseous state
Chemistry End of Section Exercises
- Heat is added to boiling water. Explain why the temperature of the boiling water does not change. What does change?
- What feature characterizes the dynamic equilibrium between a liquid and its vapor in a closed container?
- Identify two common observations indicating some liquids have sufficient vapor pressures to noticeably evaporate?
- What is the relationship between the intermolecular forces in a liquid and its vapor pressure?
- Why does spilled gasoline evaporate more rapidly on a hot day than on a cold day?
- When is the boiling point of a liquid equal to its normal boiling point?
- Explain the following observation: It takes longer to cook an egg in Ft. Davis, Texas (altitude, 5000 feet above sea level) than it does in Boston (at sea level).
- Which series below has the compounds listed correctly in order of increasing boiling points?
- N2 < CS2 < H2O < KCl
- H2O < N2 < CS2 < KCl
- N2 < KCl < CS2 < H2O
- CS2 < N2 < KCl < H2O
- KCl < H2O < CS2 < N2
Answers to Chemistry End of Section Exercises
- The heat is absorbed by the boiling water, providing the energy required to partially overcome intermolecular attractive forces in the liquid and causing a phase transition to gaseous water. The solution remains at 100 °C until all the water is vaporized. Only the amount of water existing as liquid changes until the liquid disappears. Then the temperature of the gaseous water can rise.
- Vapor pressure
- We can see the amount of liquid in an open container decrease and we can smell the vapor of some liquids.
- The vapor pressure of a liquid decreases as the strength of its intermolecular forces increases.
- At higher temperatures, the average kinetic energy of gasoline molecules are higher and therefore a greater fraction of molecules have sufficient energy to escape from the liquid than at lower temperatures.
- When the pressure of gas above the liquid is exactly 1 atm.
- At 5000 feet, the atmospheric pressure is lower than at sea level, and water will therefore boil at a lower temperature. This lower temperature will cause the physical and chemical changes involved in cooking the egg to proceed more slowly, and a longer time is required to fully cook the egg.
- a