M2 | Spinal Mechanisms for Sensorimotor Integration
Somatosensory pathways
Functional organization of spinal and cranial nerves
The CNS receives somatosensory information via 31 spinal nerves that originate from cervical, thoracic, lumbar, and sacral segments of the spinal cord, and 12 cranial nerves that originate from the brainstem. Spinal nerves enter the CNS through openings between adjacent vertebrae; cranial nerves enter the CNS through openings in the cranium.
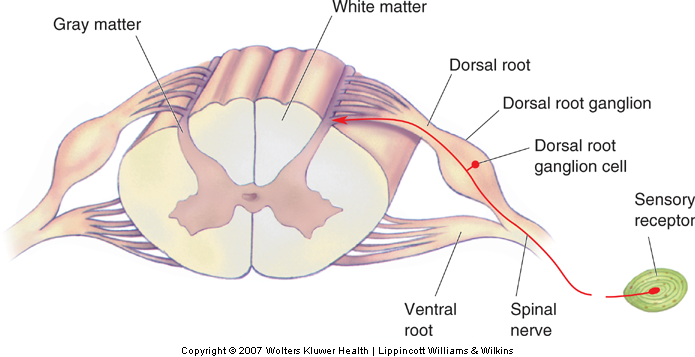
The cell bodies of somatosensory neurons are located in the dorsal root ganglia of the spinal cord (or in the cranial ganglia of the brainstem). The axon of a somatosensory neuron bifurcates; its peripheral branch innervates the sensory receptor and its central branch transmits action potentials from the sensory receptor into the spinal cord (or brainstem). There is a dorsal root (or cranial) ganglion for each spinal (or cranial) nerve. Most spinal and cranial nerves are mixed nerves, that is, they carry both afferent (somatosensory) as well as efferent (motor) signals.
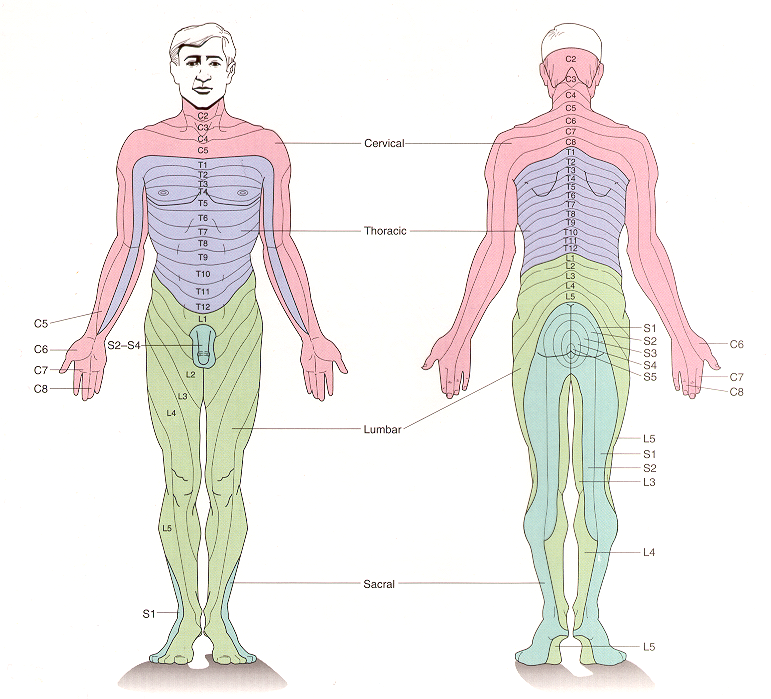
Each peripheral nerve carries somatosensory afferents that originate in the skin or deep tissues of a particular region of the body. The region of skin innervated by a single spinal or cranial nerve is called a dermatome. Dermatomal maps are significant clinically as they permit localization of injuries that give rise to restricted sensory deficits.
Functional organization of ascending somatosensory pathways
Somatosensory pathways consists of chains of three neurons. The first-order neuron (primary sensory afferent) innervates the receptor, has a cell body in the dorsal root (or cranial) ganglion, and an axon that terminates in the spinal cord (or brainstem). The second-order neuron has its cell body in the spinal cord (or brainstem) and terminates in the thalamus. The third order neuron has its cell body in the thalamus and its axon terminates in the primary somatosensory cortex.
Overview
Two main pathways transmit somatosensory signals from receptors to the primary somatosensory cortex: 1) the dorsal columns-medial lemniscus and 2) the spinothalamic tract. Both pathways carry modality-specific information. The dorsal columns-medial lemniscus transmits information of discriminative touch; the spinothalamic tract transmits information of nociception (pain) and temperature. Both somatosensory pathways are crossed, that is, receptors on the right side of the body transmit information to the left thalamus and primary somatosensory cortex, and vice versa. Both pathways are also somatotopically organized, that is, the body is represented in an orderly fashion within each of the two pathways.
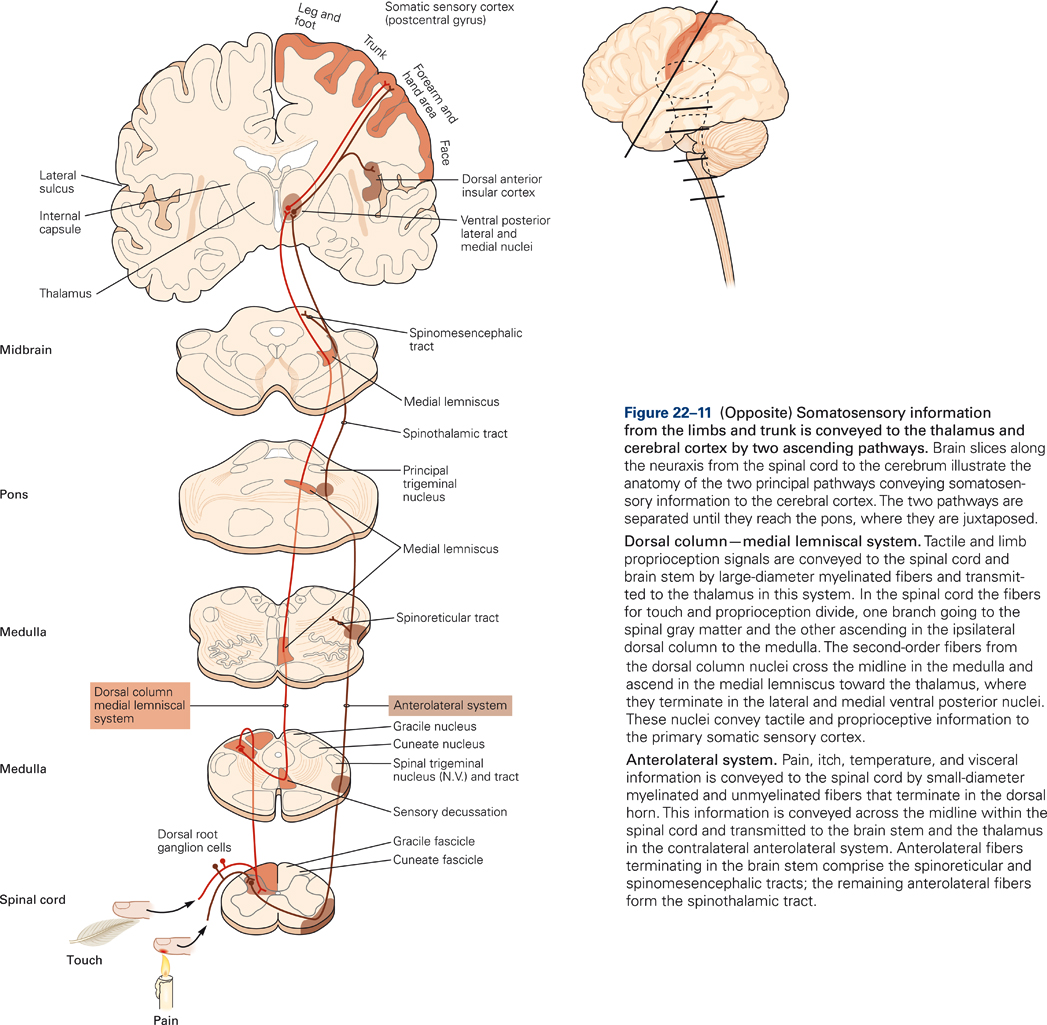
Dorsal columns – Medial lemniscus system
Primary afferents with the thickest axon diameters carry information from low threshold mechanoreceptors of the skin, muscles and joints. These pathways transmit fast and precise information from skin and skeletal muscles about the type of stimulus (for example, touch, vibration, pressure), the exact site of the stimulus, and information about stimulus onset and offset. Thus, the information consists of the what? where? and when? of discriminative sensations. Discriminative sensations are related to spatial and temporal features of the stimuli. So, for example, patients with lesions of the dorsal column pathways may still recognize a coin pressed in the palm of their hand but cannot distinguish between a larger and a smaller coin. Or they may be able to identify that something is moving along their skin but cannot identify the direction of movement. They are not aware about where their limbs are in relation to their body. Discriminative sensation is very important for performing voluntary movement. Without it, movements become severely ataxic, that is, uncoordinated and insecure. Especially movements that require feedback from the periphery are impaired. Typically, patients with damage to the dorsal columns rely more heavily on visual information. Movements for which visual information cannot compensate for the loss of somatosensory information are most severely impaired (for example, buttoning cloths).
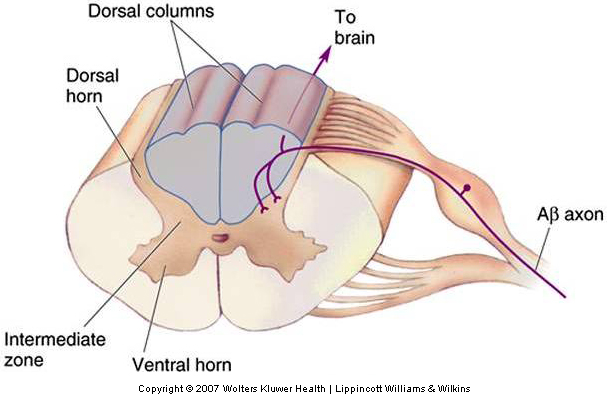
The large diameter myelinated fibers carrying touch and proprioceptive information ascend ipsilaterally (i.e. on the same side of the body as the receptor) in the dorsal columns and synapse on secondary neurons in the dorsal column nuclei in the medulla (Fig. 22-11). The axons of the dorsal column neurons cross the midline and form the medial lemniscus, a fiber tract that ascends through the medulla, pons, and midbrain and terminates in the ventral posterior (VP) nuclei of the thalamus.
Spinothalamic tracts
The small diameter Aδ and C fibers carrying information about pain and temperature (and crude information about touch and pressure) synapse on cells lateral in the dorsal horn of the spinal cord. Cells differ in their response characteristics. For example, there are low threshold, high threshold, and wide dynamic range neurons. Wide dynamic range neurons respond to both low- and high-threshold stimuli.
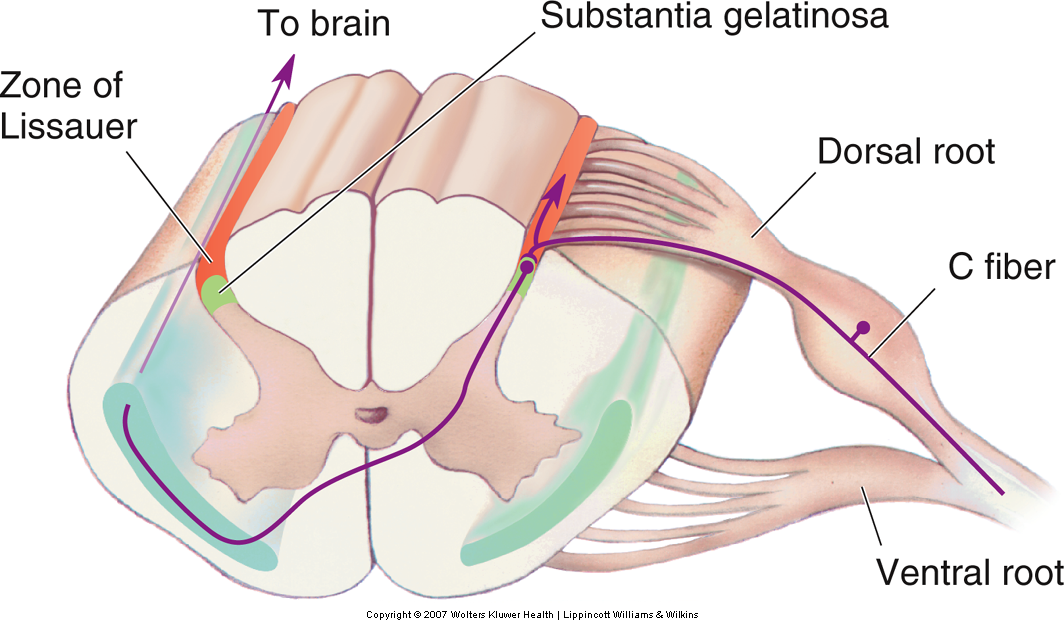
In contrast to the dorsal column-medial lemniscal pathway, the axons of the secondary neurons cross the midline at the same spinal segmental level as they enter, and they ascend contralaterally in the spinothalamic tract. The spinothalamic tract is located in the ventrolateral columns of the spinal cord and terminates in the thalamus (Fig. 22-11). Thus, touch and proprioceptive information ascends ipsilaterally in the spinal cord whereas pain and temperature information ascends contralaterally. Therefore, a spinal hemi section, for example, will produce deficits in touch and proprioception on the same side of the body but deficits in pain and temperature sensation on the opposite side of the body.
Thalamus
The ventral posterior (VP) thalamus is a critical relay for the flow of sensory information to cerebral cortex. Although generally referred to as a relay, in recent years it has become apparent that the thalamus is a site of significant signal processing in both the dorsal column-medial lemniscal (DC-ML) as well as spinothalamic systems. Inhibitory interactions between adjacent input neurons of the DC-ML system, for example, form the basis for lateral inhibition, which enhances tactile sensitivity. Furthermore, descending projections from cerebral cortical areas target both thalamic and dorsal column nuclei and play a role in the gating of sensory signals by motor output.
The medial part of VL thalamus receives trigeminal fibers from the head and face; the lateral parts receive inputs from the dorsal column nuclei. These inputs are arranged somatotopically, with fibers from the forelimb terminating medially and trunk and hindlimb laterally. Thalamic subdivisions that process the various submodalities (touch, proprioception, nociception) are largely non-overlapping, as are their target areas in the somatosensory areas of the cerebral cortex.
Somatosensory areas of cerebral cortex
Somatosensory information is transmitted from the thalamus to three regions in the parietal lobe: the primary somatosensory cortex (S-I) in the post-central gyrus, the secondary somatosensory area (S-II), and the posterior parietal cortex (PPC). These regions are critical for the experience of touch and for kinesthesis, the perception and conscious awareness of body position and movement.
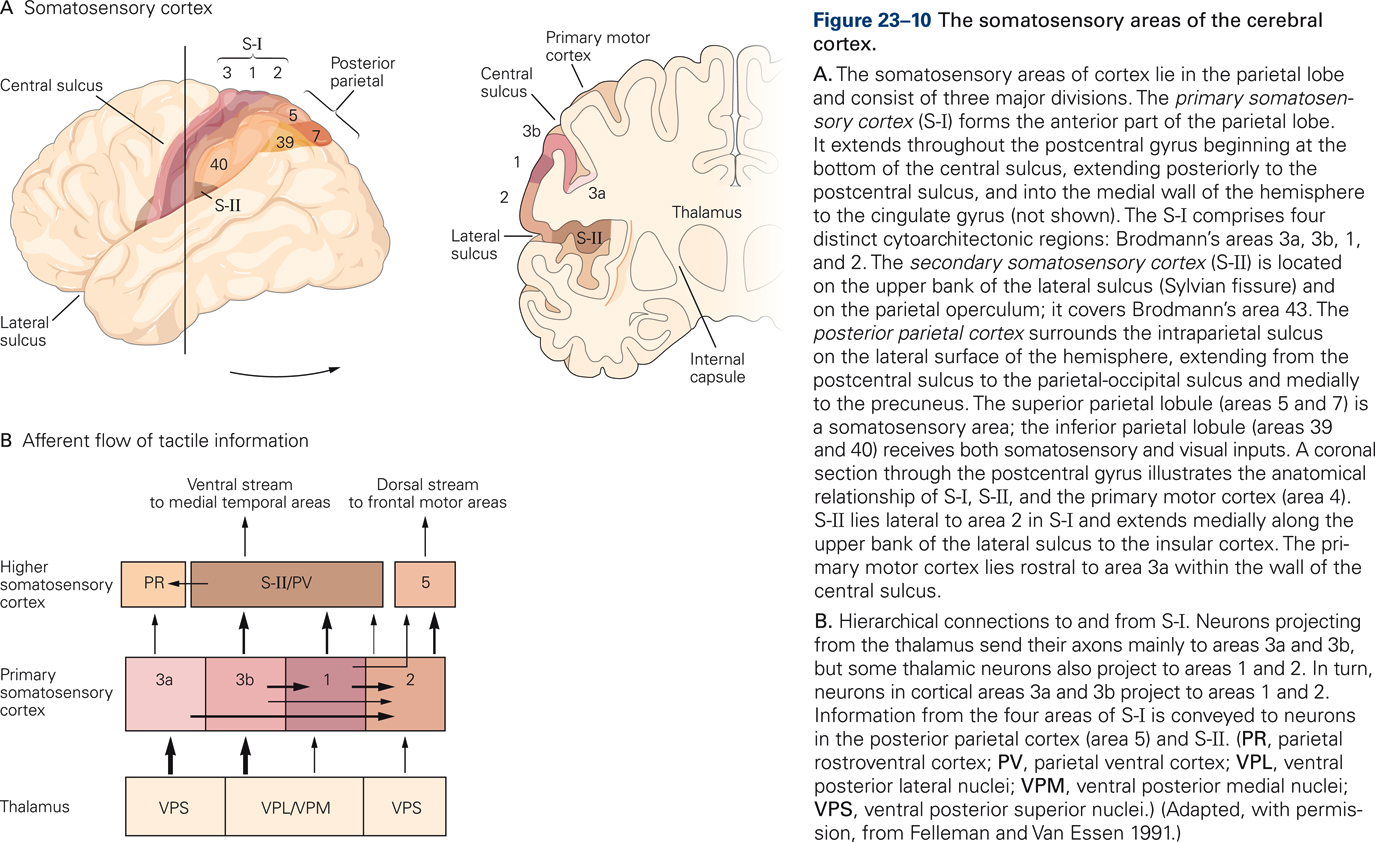
S-I has four subdivisions: areas 3a, 3b, 1, and 2. These so-called Brodmann’s areas were originally distinguished on the basis of cytoarchitectonics such as cell size, cell density, relative thickness of cortical layers, etc. (Brodmann, 1909) but were later found to also be distinct in function. Areas 1 and 3b process signals from primarily cutaneous afferents whereas areas 3a and 2 process signals from muscle and joint afferents. Somatosensory cortices are arranged hierarchically: the four areas of S-I transmit information to higher somatosensory cortices S-II and PPC.
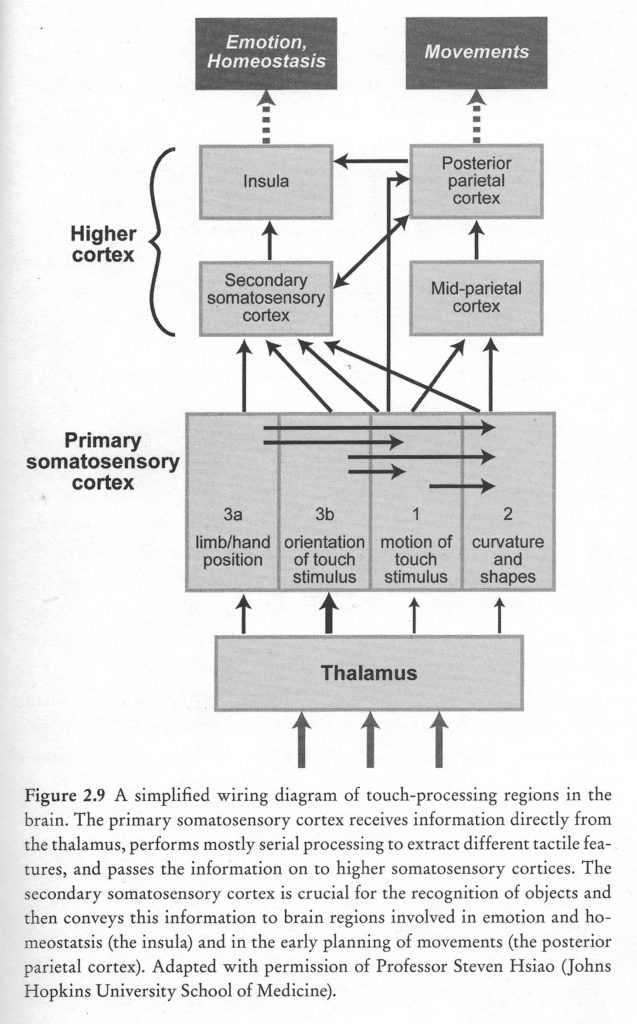
Cortical receptive fields
Receptive fields of cortical neurons are typically larger in size than those of peripheral somatosensory receptors, reflecting that cortical neurons integrate information from nearby receptors. Cortical neurons in areas 1 and 2 receive convergent inputs from different types of somatosensory receptors that innervate the same body part. Receptive fields of somatosensory neurons in higher cortical areas may comprise regions of skin that are defined functionally, such as regions that are activated simultaneously by specific hand movements, for example, several fingers in addition to the palm of the hand. Neurons in area 5 are activated by active touch, i.e. when manipulating objects. Receptive field of neurons in S-II and PPC often respond to touch at mirror image locations on both hands, suggesting a role in bilateral coordination.
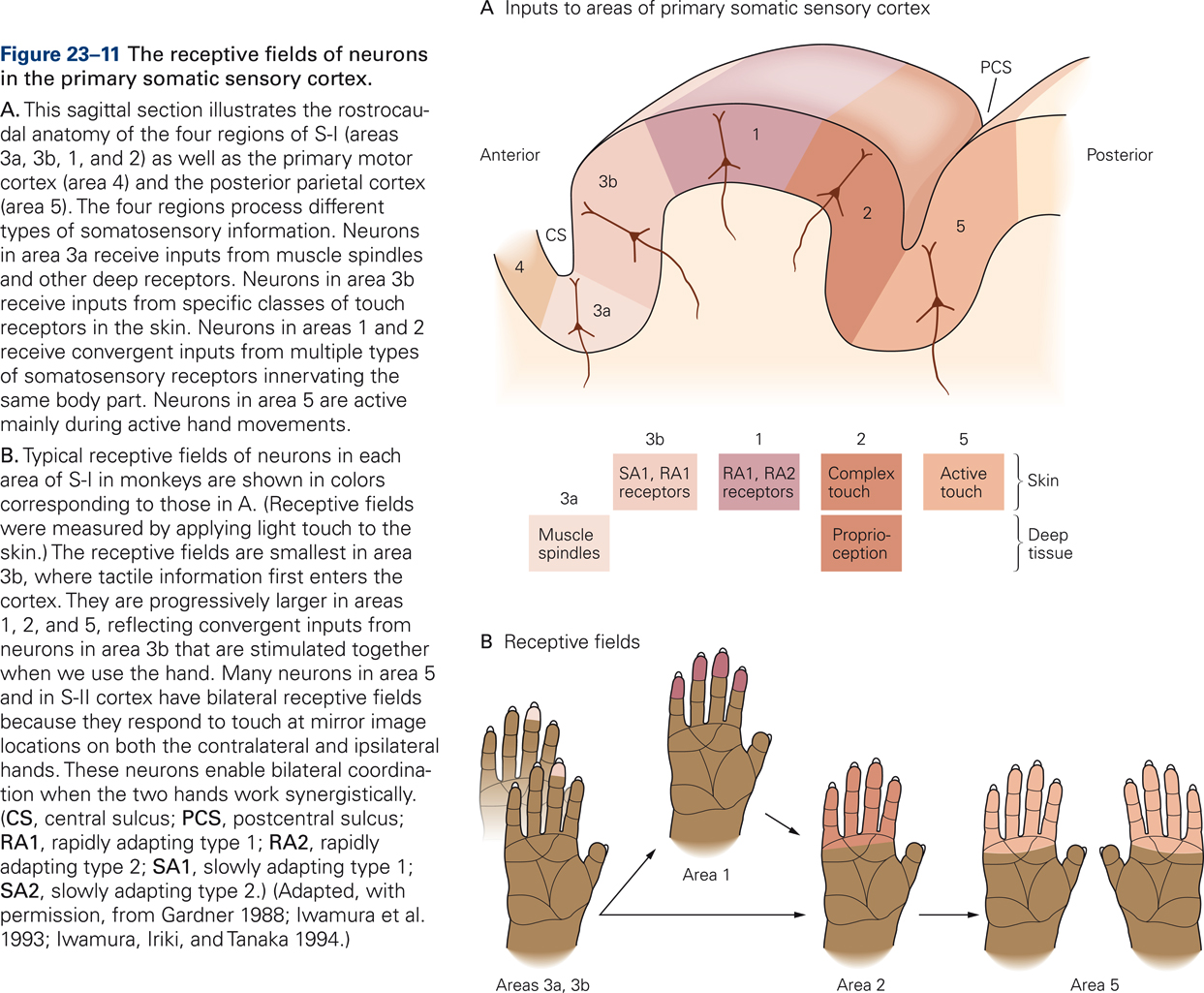
Primary somatosensory cortex (S-I) is critical for tactile discrimination. Responses of S-I neurons to touch are dominated by ascending inputs carrying information from peripheral receptors. In keeping with this view, firing patterns of neurons in S-I encode detailed spatial and temporal features of tactile stimuli with high fidelity. In contrast, in higher somatosensory cortices, touch information is encoded in more abstract form, and firing patterns are influenced by behavioral context and motivational state. S-II neurons, for example, play a key role in processing past and present sensory information that encodes spatial features of stimuli, such as shape and texture, in order to recognize objects placed in the hand (Romo, Hernandez, Zainos, Lemus, & Brody, 2002).
Posterior parietal cortices (PPC, areas 5 and 7) are not directly involved in tactile discrimination but, instead, are critical for sensory guidance of movement (Mountcastle, 1995). Area 5 neurons discharge strongly during active touch, object manipulation, and when monkeys shape and extend their hand during reach-to-grasp movements (Gardner et al., 2007). Area 7 neurons integrate tactile and visual information and play a role in eye-hand coordination (ref).
In order to successfully interact with our environment and achieve our behavioral goals, we need motor prediction, which requires the ability to compare planned and actual movements. For example, reaching to grasp moving objects requires motor commands that accurately predict the location of the object at the end of the reach. The posterior parietal cortices and associated circuitry may represent neural substrates that underlie motor prediction. Convergence of central and peripheral inputs in posterior parietal cortices may serve to compare sensory consequences of movements, predicted on the basis of motor commands, with actual sensory feedback that becomes available as the movement is executed (Blakemore & Sirigu, 2003; Wolpert & Flanagan, 2001). Discrepancies in predicted and actual sensory feedback may be used to distinguish active (self-generated) from passive movements, and may serve as error signals for necessary corrective actions.
Somatotopic organization
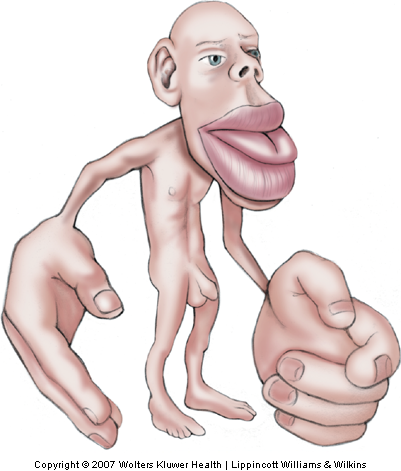
Somatotopic organization refers to a topography that preserves the spatial relations of receptors on the body surface across successive levels of neural processing. Somatotopic organization gives rise to an orderly representation of the body within a neural structure or circuit. The primary somatosensory cortex (S-I) offers one of the finest examples of a somatotopy (Penfield & Rasmussen, 1950)(Fig. 12-19). Each of the four areas (3a, 3b, 1, and 2) of S-I contains a complete map of the body, with the leg represented medially and the hand and face represented progressively more laterally (Nelson, Sur, Felleman, & Kaas, 1980; Pons, Garraghty, Cusick, & Kaas, 1985). Knowledge of somatotopic organization is important for localization of damage following stroke or traumatic brain injury.
The volume of brain tissue devoted to processing touch information from different parts of the body varies over a wide range, according to the innervation density of receptors in a given area of skin (Sur, Merzenich, & Kaas, 1980) and, thus, reflects the relative importance of a given body part for the sense of touch. Disproportionally large volumes of tissue are devoted to the hand, foot, and mouth, and relatively smaller volumes to the trunk. This unequal distribution gives rise to the well-known distorted ‘homunculus’ with enormous size hands and lips and a relatively much smaller torso (Fig. 12-20).
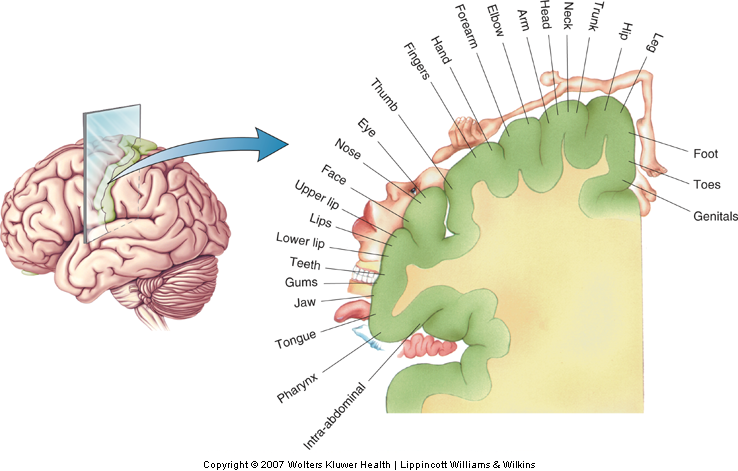
Neural plasticity
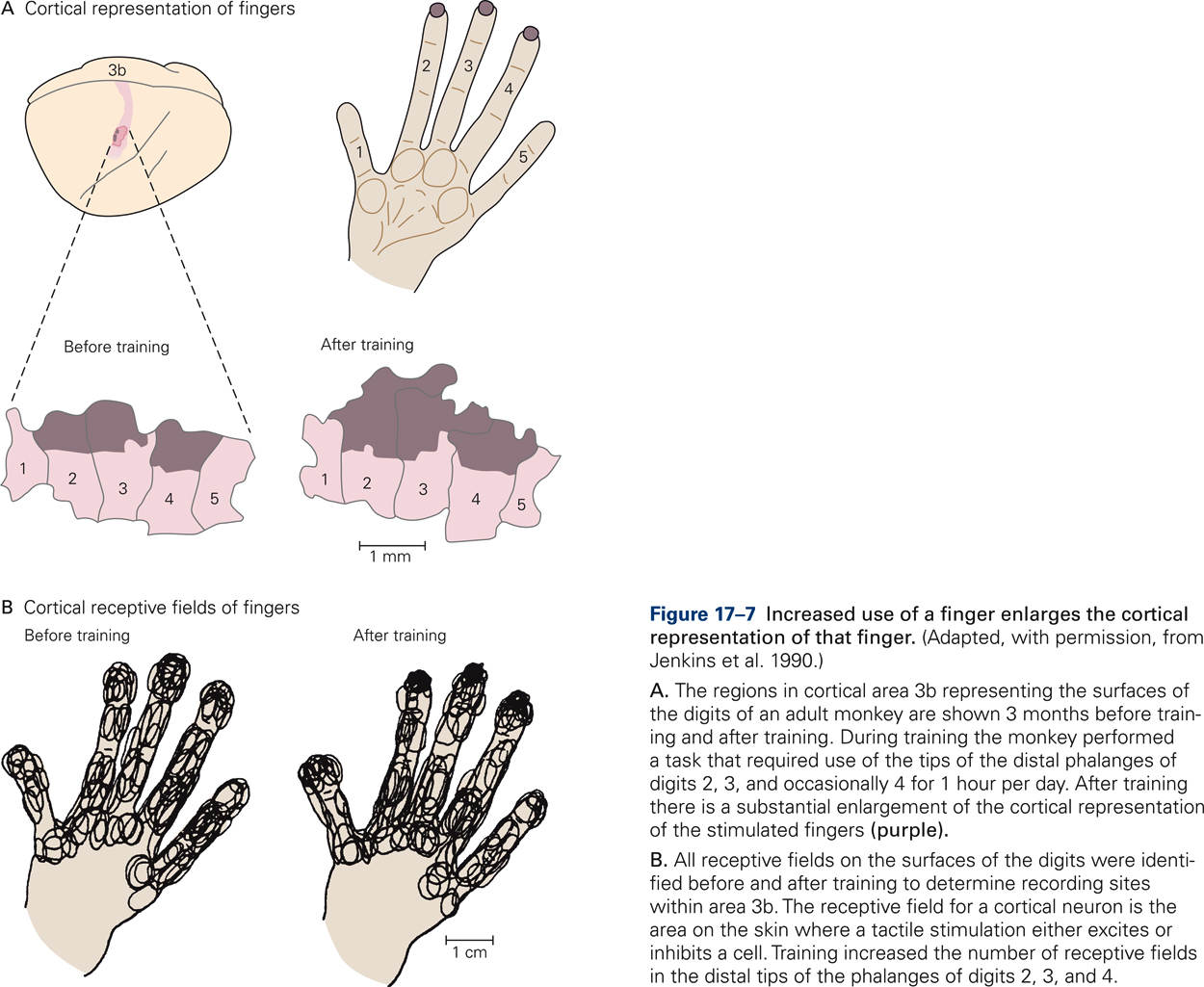
The somatotopic organization within primary somatosensory cortex is not fixed but is dynamic. Genetic blueprint plays an important role in establishing the basic somatotopic organization of S-I but genes must interact significantly with environmental influences to determine the exact layout. The somatotopic mapping is plastic and adjusts depending on experience. This so called neural plasticity is a key feature of brain structure and function and forms the basis for neurorehabilitation by physical and occupational therapy following neurological injury or disease. Information about how the brain maps with experience may also inform and guide the development of educational programs.
Evidence that somatotopic maps are not hard-wired and that experience can change the layout was provided by experiments in which owl monkeys were trained to touch a rotating disk with the tips of their middle fingers in order to get food rewards (Jenkins, Merzenich, Ochs, Allard, & Guic-Robles, 1990). After several months of training, the cortical territories corresponding to the tips of the middle fingers were greatly enlarged relative to the territories of the tips of the adjacent fingers, which did not receive differential stimulation during the training period (Fig. 17-7A). In addition, training increased the number of receptive fields in the distal fingertips of the differentially stimulated digits (Fig. 17-7B). The results demonstrate that increased use of a finger enlarges the cortical representation of that finger and can strengthen the neural connectivity along the pathways that connect the peripheral receptors in the skin with the somatosensory cortex.
Neural plasticity also underlies sensory substitution. Consider for example that most of us read using visual information; however, some people are extremely proficient in reading with their fingertips, a feat that is truly remarkable! Society regards this population as disabled or visually-impaired; however, blind persons outperform ‘visually-abled’ individuals in somatosensory discrimination tasks by substituting somatosensory for visual information. Additionally, blind persons repurpose and adapt neural circuitry for processing somatosensory rather than visual information. Neural adaptations that underlie these phenomena are just beginning to be revealed as a result of advances in technology that make it possible to study neural plasticity non-invasively.
Studies of organization of sensory cortices in people that are congenitally blind or deaf have demonstrated that the extent of brain plasticity is substantial (C. Karns, 2012). When a major sense is absent from birth, the sensory cortices adapt, processing information from intact senses. For example, the primary auditory cortex responds to touch and vision in congenitally deaf adults, significantly more so than in hearing people (C. M. Karns, Dow, & Neville, 2012).