Unit Two
Day 13: Alcohols, Carboxylic Acids, Amines, Amides; Hydrogen Bonding
D13.1 Alcohols
An alcohol functional group contains a –OH (hydroxyl) group covalently bonded to a carbon atom. (Note that the –OH group is covalently bonded—the OH is not a hydroxide ion.) The names of alcohols are derived from the names of the corresponding alkanes by removing the final “e” and adding “ol”. For example, the structure of the simplest alcohol is derived from the structure of methane by replacing one H atom with an OH group (CH3OH). It is named methanol .
Ethanol is produced by some species of yeast that catalyze fermentation of various sugars:
Ethanol can also be synthesized using the addition reaction of ethene and water using an acid as a catalyst:
Exercise 1: Structures of Alcohols
Knowing how to systematically name compounds can be helpful, especially when trying to decipher whether the line structures shown are constitutional isomers or not. See the nomenclature appendix for additional descriptions on how to name alcohol compounds. (You will not be explicitly tested on nomenclature in this course.)
Alcohol molecules can be classified according to the number of alkyl groups attached to the carbon with the -OH group, as shown in Figure 1. If one alkyl group (or only hydrogen) is attached to that carbon, the alcohol is a primary (1º) alcohol. If two alkyl groups are attached, the alcohol is a secondary (2º) alcohol. If three alkyl groups are attached, the alcohol is a tertiary (3º) alcohol.
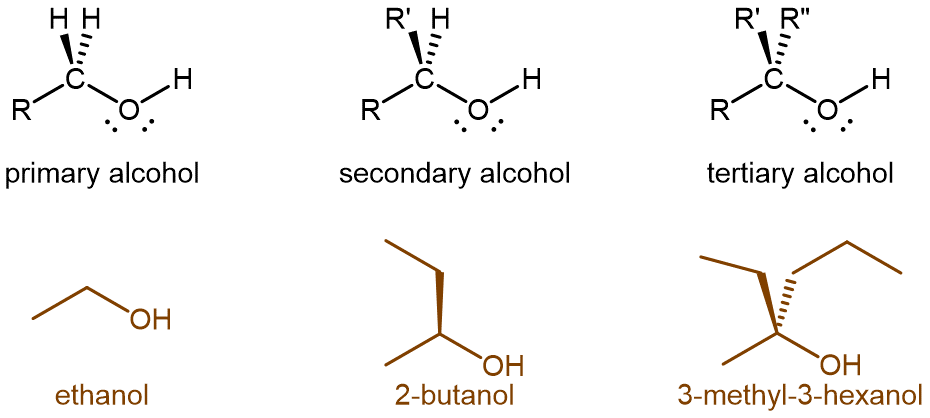
D13.2 Carboxylic Acids
A carboxylic acid functional group, -COOH, has a carbonyl and a hydroxyl (-OH) group linked to the same carbon atom. It differs from an ester in that the non-carbonyl oxygen is bonded to a hydrogen atom rather than an R group. Hence, carboxylic acid groups are found at one end of a molecule.
The simplest carboxylic acid is formic acid, known since 1670. Its name comes from the Latin word for ant, formicus. It is partially responsible for the pain and irritation of ants’ and wasps’ stings. Acetic acid is a main component (>4% by volume) of vinegar. Cider vinegar is produced by allowing apple juice to ferment without oxygen present; yeast changes sugar to ethanol, which is then converted to acetic acid via biological oxidation. Butyric acid, a component of rancid butter and Limburger cheese, has a vile odor.
Exercise 2: Identifying Alcohols from Structure
Carboxylic acid groups are acidic because their O-H bond can break relatively easily to yield -COO– and H+, allowing them to donate a proton, H+, to another molecule or ion. This acidity arises from the relative stability of the carboxylate anion, RCOO−.
Activity 1: Resonance and Acidity of a Carboxylic Acid
D13.3 Amines
An amine functional group is a derivative of ammonia that contains one or more carbon-nitrogen bonds.
You can classify amine molecules by the number of C-N bonds. In the above example, methylamine is a primary (1º) amine, dimethylamine is a secondary (2º) amine, and trimethylamine is a tertiary (3º) amine. In this figure methyl groups are shown but any R group, such as ethyl, could replace any of the methyl groups.
Like ammonia, amines are basic due to the lone pair on the nitrogen atom, and can undergo acid-base reactions to form protonated amine cations analogous to the ammonium ion NH[latex]_4^+[/latex]:
D13.4 Amides
An amide functional group contains a nitrogen atom connected to the carbon atom of a carbonyl group. Like amines, amides can be classified by the number of carbon atoms bonded to the nitrogen:
Activity 2: Amines, Amides, and Resonance Structures
Although the resonance structure with formal charges on O and N does not contribute as much to the resonance hybrid as the resonance structure without formal charges, it is crucial in understanding the chemical and physical properties of amide molecules. For example, the partial double bond character gives rise to a significant energy barrier for rotation of the C-N bond. And because the lone pair on the N atom is part of the π bonding network, the N atom in an amide is about 1010 times less basic than the N atom in an amine.
Exercise 3: Structures of Amines
D13.5 Reactions of Alcohols, Amines, and Carboxylic Acids
Oxidation Reactions
All substances whose molecules contain hydrocarbon sections are combustible—the hydrocarbon parts can be oxidized completely to carbon dioxide and water. For many compounds controlled oxidation is more important than combustion because it can convert one type of functional group into another functional group, giving us chemical compounds useful in various applications. An example is oxidation of alcohols, which can be converted to aldehydes, ketones, or carboxylic acids.
Oxidation of an organic compound can usually be recognized as either an addition of oxygen atom(s) to or removal of hydrogen atoms from the reactant molecule. The ease with which an alcohol can be oxidized and the extent of the oxidation depends on whether the alcohol is primary, secondary, or tertiary.
For a primary alcohol, controlled oxidation first produces an aldehyde. If there is an excess of oxidant, the aldehyde is further oxidized to a carboxylic acid:
Common oxidizing agents used in the laboratory for controlled oxidation are aqueous solution of potassium permanganate, KMnO4(aq), or aqueous acidic potassium dichromate, K2Cr2O7(aq).
For a secondary alcohol, the oxidation product is a ketone:
Ketones are difficult to oxidize further because, without breaking C–C bonds, there is no obvious way to add one more oxygen atom to the carbonyl carbon and there are no hydrogen atoms to remove from that carbon either.
Tertiary alcohols, with no hydrogen atoms attached to the carbon atom that is bonded to the –OH group, are difficult to oxidize. Tertiary alcohols do undergo combustion (to yield CO2 and H2O), but they usually do not undergo controlled oxidation.
Condensation Reactions
In a condensation reaction, two molecules join to form a larger molecule and a small, stable molecule such as H2O or HCl. For example, ethyl acetate, CH3COOCH2CH3, is formed when acetic acid reacts with ethanol in the presence of an acid catalyst:
In general, condensation of a carboxylic acid and an alcohol produces an ester.
Condensation reactions are reversible: not all reactant molecules are converted to product molecules and, if product molecules are mixed, some reactant molecules form. The reverse reaction of a condensation that produces water is called a hydrolysis reaction. In hydrolysis, water breaks apart into H and OH with H attaching to one part the larger molecule and OH to another: hydrolysis comes from hydro, “water” and lysis, “breaking apart”.
Ethers can be obtained from condensation reactions involving two alcohol molecules. If the two alcohol molecules are the same, a symmetric ether forms. For example, when ethanol is treated with a limited quantity of sulfuric acid and heated to 140 °C, diethyl ether and water are formed:
If the condensation reaction involves two different alcohols, an asymmetric ethers can form. For example:
Alternatively, addition reactions between alkenes and alcohols can form ethers. For example:
Amides can be produced from carboxylic acids and primary or secondary amines (or ammonia) via condensation reactions:
It is through this reaction that amino acids (molecules containing both an amine and a carboxylic acid functional group) link together in a polymer to form peptides and proteins.
Exercise 4: Recognizing Functional Groups
Applying Core Ideas: Comparing Propane, Dimethyl Ether, and Ethanol
When we previously compared the boiling points of propane and dimethyl ether, we saw the effect of dipole-dipole attractions. Let’s add ethanol to that comparison:
D13.6 Intermolecular Forces: Hydrogen Bonding
The additional IMF that exists between ethanol molecules, but does not exist between propane molecules or between dimethyl ether molecules, is called hydrogen bonding. It is the interaction between an X–H covalent bond (X denotes a highly electronegative atom) and the lone pair on an electron rich atom, Z. The hydrogen bonding interaction can be designated as X-H···Z (the “···” is the hydrogen bond itself).
Strong hydrogen bonding occurs between F-H, O-H, or N-H bond and an electron lone pair on another F, O, or N atom. F, O, and N, are among the most electronegative elements in the periodic table, a characteristic necessary for strong hydrogen bonding.
A hydrogen bond has about 5-10% the strength of a typical covalent bond. Part of this strength comes from dipole-dipole interactions, but more important is partial electron sharing that resembles the formation of a covalent bond. This is illustrated in Figure 2, which shows the hydrogen bond interaction between two water molecules. The left water molecule provides the O-H bond (we will denote this as OL-H); the right water molecule provides the O atom with the lone pair (we will denote this as OR).
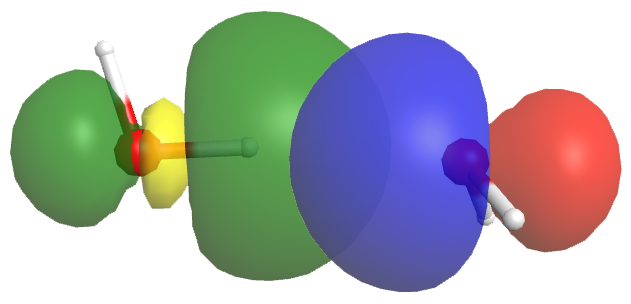
The empty OL-H antibonding σ* orbital (yellow/green) overlaps with the orbital of the OR atom’s lone pair (blue/red). This overlap allows some electron density to be shared between the two water molecules, thus forming a hydrogen bond. If more electron density were to move from the OR lone pair to the OL-H σ* orbital, the antibonding orbital would be filled, the OL-H bond would break, and a chemical reaction would have occurred—a new OR-H bond would form, resulting in the right water molecule becoming H3O+:
Thus, forming a hydrogen bond resembles the formation of a covalent bond.
Activity 3: IMFs between Water Molecules and between Hydrogen Fluoride Molecules
For amines, 1º and 2º amines are capable of strong hydrogen bonding due to the presence of a N-H bond and the lone pair on the N atom, but 3º amines cannot form hydrogen bonds. For example, for the three amine isomers shown below, the boiling point of the 3º amine is significantly lower than the 1º and 2º isomers.
For amides, because the lone pair on N atom is part of the π bonding network, it is not available for hydrogen bonding. However, hydrogen bonds can still form in 1º and 2º amides between the N-H bond(s) and the lone pairs on the O atom.
Molecules containing the carboxylic acid functional group can also form hydrogen bonds. Pure acetic acid is called glacial acetic acid because its melting point of 16.6 °C is high enough that it can freeze in a cold laboratory. It is also quite thick and syrupy because the many hydrogen-bonding attractions between molecules result in high viscosity. The acidity of the carboxylic acid group enhances the O-H···O hydrogen-bond strength, such that hydrogen-bonding between carboxylic acid molecules is usually greater than between alcohol molecules. For example:
D13.7 Intermolecular Forces: Water Solubility
IMFs directly affect the water-solubility of a compound. Let’s consider the alcohol functional group as an example.
The -OH end of an alcohol molecule is hydrophilic (“water-loving”), capable of strong intermolecular interactions with water molecules. The dipole-dipole and hydrogen-bond interactions between alcohol and water molecules are similar in strength to those between alcohol and alcohol molecules or between water and water molecules. The hydrocarbon (alkyl) end of an alcohol molecule is essentially nonpolar and is hydrophobic (“water-fearing”), where the intermolecular interactions between hydrophobic molecules and water molecules are weak compared to IMFs between water-water molecules or hydrocarbon-hydrocarbon molecules.
Hence, although the size of the alkyl group has little influence on the reactivity of an alcohol molecule, it has a significant impact on the solubility of alcohols in water. Alcohols with small alkyl groups, e.g. methanol and ethanol, are completely miscible with water, which means they have infinite mutual solubility in water. Alcohols with larger alkyl groups, such as 1-octanol, are immiscible in water, which means that when 1-octanol is added to water two layers form. One layer is nearly pure water and the other layer nearly pure 1-octanol, because the solubility of each substance in the other is very low.
This is similarly true for the other polar and hydrogen-bonding functional groups. For example, acetic acid is miscible with water, while octanoic acid is immiscible with water.
Day 13 Pre-class Podia Problem: Functional Groups, Reactions, Solubility
Consider the structures shown below. List all substances that fall into each of the five categories below and explain why each substance fits each category you placed it in. Also explain why the other substances do not fit each category.
- Contains a secondary alcohol functional group.
- Is less soluble in water than 1-hexanol.
- Has a higher boiling point than 1-hexanol.
- Reacts with K2Cr2O7(aq) to form a carboxylic acid.
- Undergoes addition of water (with an acid catalyst) to form a secondary alcohol.
Two days before the next whole-class session, this Podia question will become live on Podia, where you can submit your answer.