Unit One
Day 1: Chemistry, Matter, Energy, Models
Welcome to Chemistry 109!
If you have not yet worked through the Introduction, please do so before beginning this section.
Today’s work begins with a review of important fundamentals of chemistry. We expect that you are familiar with these ideas and are ready to use them to help you learn more chemistry. Then we introduce fundamental ideas about energy and matter. These ideas will recur throughout this course and we expect they will serve you well during your entire career
D1.1 Substances and Chemical Reactions
A fundamental aspect of chemistry is that substances change into other substances. A process in which one or more substances changes into one or more different substances is called a chemical reaction. This course aims to enhance your understanding of substances and chemical reactions. For a specific substance, what properties can you expect? Given one or two substances, is a reaction likely? When a reaction occurs, what new substances are formed? How is energy related to chemical reactions? Can we make new substances that have properties we want (such as alleviating disease)? Ability to answer such questions is valuable in a broad range of fields, from physics to pharmacy.
Activity 1: Chemical Reactions
Reactions of copper with air and of copper oxide with hydrogen:
Saying that one substance changes into another is not very precise without a definition of what a substance is. In chemistry, a substance is matter that, when purified, has specific, characteristic properties and composition. For example, all samples of pure copper have an orange, lustrous surface; all conduct electricity equally well; all react with dry air to produce a black substance; and all consist solely of copper. The American Chemical Society currently lists more than 160 million chemical substances in a data base. New substances are being synthesized every day.
Because there are so many chemical substances, it is useful to develop categories and classifications to help guide our thinking. The most important is that chemical substances are composed of only a few chemical elements (118 of which have been discovered so far). A chemical element is a substance that cannot be changed by chemical reaction into two or more different substances. Elements combine to form chemical compounds, substances that can be decomposed by chemical reactions into two or more new substances. If you know something about the chemical elements and how they combine to form chemical compounds, you can predict properties of a wide range of substances and often predict which substances are likely to react to form what products.
Another useful classification is to divide the elements into metals and nonmetals. Metals conduct electricity as both solids and liquids, have lustrous surfaces when pure, can be pounded into different shapes and drawn into wires, and conduct heat well. Nonmetals have very small electrical conductivity and have a broad range of other properties. Six elements, the metalloids, have properties intermediate between metals and nonmetals.
Chemical reactions and the classifications of substances discussed so far are based on what are called macroscopic observations. Macroscopic refers to things large enough to be seen and manipulated in a laboratory (or anywhere else). Enhancing your understanding of the macroscopic world is a goal of this course.
D1.2 Atoms, Molecules, and Ions
A different fundamental aspect of chemistry is that we can better understand elements, compounds, and reactions if we build models based on tiny particles that are constantly in motion: atoms and molecules.
An atom is the tiniest particle of an element that has the chemical properties of that element. Atoms are extremely small, with sizes on the order of 100 pm to 350 pm (1 pm = 10−12 m). The smallest thing discernible with the most powerful optical microscope is more than 1000 times bigger than an atom. A molecule is two or more atoms connected by chemical bonds. Atomic scale or sub-microscopic scale refers to things about the same size as atoms and molecules. Chemists build atomic-scale models to interpret and predict macroscopic phenomena. For example, many substances consist of molecules; the properties of these molecular substances can be predicted if we know which atoms make up their molecules and how those atoms are arranged.
Atoms contain three kinds of particles: protons, neutrons, and electrons. Atoms are identified by the number of protons in the nucleus, the atomic number. Protons are positively charged. Neutrons have no electric charge. Electrons have negative charge with the same magnitude as a proton’s positive charge. Protons and neutrons have much greater mass than electrons and are found in the nucleus, a very small volume in the center of an atom that contains most of the atom’s mass. Electrons, which constitute less than 1/1000 the mass of an atom occupy 99.9999999999999% of the space the atom occupies. When a chemical reaction occurs, atoms remain unchanged, except that a few electrons in the outer part of one atom may transfer to the outer part of another atom. Before and after any chemical reaction the same number of atoms of each type is present; this is known as the law of conservation of matter. When copper metal reacted with oxygen from air to form copper oxide in the first video in Activity 1, the copper oxide included all the copper atoms that originally were in the copper that reacted. When hydrogen reacted with the copper oxide those copper atoms remained on the surface as copper metal.
Ions can form from atoms or molecules. An ion is an atom or molecule that has gained or lost one or more electrons and therefore has a negative or positive electric charge. For example, when copper reacts with oxygen, the product consists of copper(II) ions and oxide ions. In a copper(II) ion a Cu atom has lost two electrons to form an ion with two units of excess positive charge, Cu2+; in an oxide ion an O atom has gained two electrons to form an ion with two units of excess negative charge, O2−. When a molecule gains or loses electrons, polyatomic ions form: the molecule NO2 forms both a positive ion, NO2+ and a negative ion, NO2−, by loss and gain of one electron.
D1.3 Chemical Symbols, Formulas, and Equations
It is convenient to deal with macroscopic properties and atomic-scale models by defining symbols to represent elements, compounds, atoms, molecules, and structures. For example, the symbol Li can represent the element lithium or it can represent a lithium atom. When Li is used to represent the element, it should bring to mind various properties: Li is a metal that can be cut with a knife; Li reacts fairly quickly with air; Li reacts vigorously with water. When Li is used to represent a lithium atom, we can use numbers to indicate how many Li atoms are present. For example, 2 Li represents two lithium atoms. In Li2O, the formula for one substance that forms when lithium reacts with oxygen, the subscript “2” indicates that there are two Li atoms for every one O atom. Li2O also represents the macroscopic substance lithium oxide, which has specific properties including high melting point (1438 °C) and high solubility in water.
Symbols can also represent chemical reactions. When lithium reacts with water the chemical equation is
2 Li(s) + 2 H2O(l) → H2(g) + 2 LiOH(aq)
The letters in parentheses (s, l, g, and aq) indicate that lithium, water, hydrogen, and lithium hydroxide are solid, liquid, gas, and an aqueous solution. The chemical symbols and formulas indicate that lithium and water are reactants and hydrogen and lithium hydroxide solution are products. The coefficients indicate how much of each reactant reacts away and how much of each product forms. The quantities can be expressed on the atomic scale as two lithium atoms reacting with two water molecules to give one hydrogen molecule and two lithium ions and two hydroxide ions in solution. (Lithium hydroxide consists of lithium ions, each with one unit of positive charge, and hydroxide ions, each with one unit of negative charge.) The quantities can be scaled up by a factor of 6.02214076 × 1023 (Avogadro’s number) and the equation says that two moles of solid lithium reacts with 2 moles of liquid water to give one mole of gaseous hydrogen and two moles of lithium hydroxide dissolved in water.
For molecules, symbolism can indicate which atoms are bonded to which other atoms, what types of bonds are present, and how the atoms are arranged in three-dimensional space. These structures illustrate some of the possibilities:
The chemical formula tells only the number of each kind of atom in the molecule. The Lewis structure indicates which atoms are bonded to which. The wedge-dash structure indicates that the molecule is three-dimensional, which is shown more clearly in the ball-and-stick model. (To see and manipulate a three-dimensional ball-and-stick model, click here.) Finally, in the space-filling model the sizes of all atoms and the molecule as a whole are shown; it is clear that a chlorine atom is bigger than a carbon atom, which is bigger than a hydrogen atom. The last two models are more pictorial than symbolic, but they are still representations of something we cannot see. None of these representations is the molecule itself; all provide useful information about its properties.
Chemists use representations such as these all the time and move effortlessly from one to the other as they think about molecules. Some imagination and much experience using these symbolic representations will enable you to make predictions about properties of substances and chemical reactions.
D1.4 The Periodic Table
As you saw in the videos in Activity 1, the elements Li, Na, K, Rb, and Cs have similar properties and react with air and water in similar ways. In addition, all these elements react with fluorine, chlorine, bromine, and iodine to form similar compounds: LiF, NaF, KF, RbF, CsF, LiCl, NaCl etc. (These compounds are referred to collectively as “salts” because their properties are similar to those of table salt, NaCl.) The reaction of Na(s) with Cl2(g) to form NaCl(s) is shown in this video.
The elements Li, Na, K, Rb, and Cs are called alkali metals. The elements F, Cl, Br, and I are called halogens. (“Halogen” comes from Greek hals, halo—”salt”.) All halogens consist of diatomic molecules, such as Cl2. Another group of elements, Be, Mg, Ca, Sr, and Ba also have similar physical properties, react with air and water (but more slowly than alkali metals), and react with halogens to form compounds with formulas like BeF2, BeCl2, MgCl2, etc. Be, Mg, Ca, Sr, and Ba are known as alkaline earth metals or alkaline earths. Just before the turn of the 20th century, chemists discovered another group of similar elements: He, Ne, Ar, Kr, and Xe. All are gases that undergo almost no chemical reactions, so He, Ne, Ar, Kr, and Xe are called noble gases. (One meaning of “noble” is “unreactive”.)
Here is a list of the first twenty elements in order of increasing atomic number:
H He Li Be B C N O F Ne Na Mg Al Si P S Cl Ar K Ca
The noble gases, alkali metals, alkaline earth metals, and halogens are color coded. Notice that the colors repeat periodically—every eight elements. About 150 years ago, Russian chemist Dmitri Mendeleev recognized this periodicity and created a table with horizontal rows of elements in atomic-weight order and groups of similar elements in vertical columns. Since Mendeleev’s time many more elements have been discovered: there are 118 in the modern periodic table below. But the idea of rows (periods) across which properties of elements vary and columns (groups) of elements with similar properties remains.
Each group (column) is identified by two numbers. The alkali-metal group is group 1 or IA; the alkaline-earth group is group 2 or IIA; the halogen group is group 17 or VIIA; and the noble-gas group is group 18 or VIIIA. The first number is designated by the International Union of Pure and Applied Chemistry; the second is more commonly used in the United States.
Down the left side of the table, the periods (rows) are numbered from 1 to 7. The first period contains only two elements; the second and third periods contain eight elements each; the fourth and fifth periods each contain 18 elements; and the sixth and seventh periods contain 32 elements. (Some elements in these latter two periods have been moved to the bottom of the table so it can fit on a printed page and be big enough to read. The curved arrows show where these elements should fit.)
The table is color coded to indicate whether an element is a metal, a metalloid, or a nonmetal. Metals conduct electricity, have lustrous surfaces when pure, combine with other metals to form alloys, and are malleable and ductile. The electrical conductivity of metals increases as temperature decreases. Non-metals are electrical insulators, usually are brittle as solids but may be liquids or gases at room temperature, and combine with other nonmetals by forming covalent bonds. Metalloids are intermediate between metals and nonmetals, Metalloids have lower electrical conductivity than metals but their conductivity increases as temperature increases.
Most elements are metals (the large blue-gray area); only 20 elements are nonmetals and six are metalloids. Notice that some groups, such as groups 14 (IVA) and 15 (VA) contain nonmetals, metalloids, and metals. This means that properties (electrical conductivity, for example) of some elements in these groups are significantly different from properties of other elements in the group; that is, in some groups elements are not as similar to each other as in the alkali-metal group. In all groups, however, the formulas of compounds are similar. For example, in group 14 (IVA), all elements form oxides with formulas XO2: CO2, SiO2, GeO2, SnO2, and PbO2. (Fl, flerovium, has been formed in quantities of only a few atoms in a particle accelerator, so the formula of its oxide has not been determined experimentally.)
Similarity of chemical formulas for elements in the same periodic group involves valence, the combining power of an atom. For example, sodium has a valence of 1 and so does chlorine; therefore, sodium and chlorine form the compound NaCl in which sodium atoms and chlorine atoms combine in a 1:1 ratio. Oxygen has a valence of 2; therefore the formula for sodium oxide is Na2O—it takes two valence 1 sodium atoms to satisfy oxygen’s valence of 2. From this you should be able to predict that the valence of C (or Si, Ge, Sn, or Pb) is 4 because the formula of carbon dioxide is CO2.
D1.5 Matter, Energy, Models
In this course you will be asked to examine data and draw conclusions, to explain phenomena by applying basic principles, and to build models from which you can predict physical and chemical properties. Two important and interconnected ideas are fundamental:
- The spatial arrangement of atomic-level particles (structure) can predict macroscopic properties and chemical reactivity;
- Energies of atomic-level particles can be used to explain atomic-level structures and macroscopic energy changes.
Atomic-scale particles adopt structures with minimum energy, unless energy is transferred to them from an external source. Therefore it is useful to be able to calculate quantitatively or predict qualitatively whether one situation has higher or lower energy than another. Chemistry often involves electrically-charged atomic-scale particles, such as protons, electrons, or ions. The potential energy of two point electric charges (charges that occupy a single geometric point) can be calculated using an equation derived from Coulomb’s law:
In this equation ke is a proportionality constant equal to 8.99 × 109 J m C−2, Q1 and Q2 are electric charge values, and r is the distance between the charges. Thus, the magnitude of the potential energy of two charged particles is proportional to the size of each charge and is inversely proportional to the distance between the charges. The energy is positive if the charges of the two particles have the same sign (both positive or both negative). The energy is negative if the charges are opposite—opposite charges attract and lower potential energy is the result. The direct proportionality to electric charge and inverse proportionality to distance enable qualitative predictions: Larger opposite charges closer together result in lower energy and hence greater stability.
Exercise 1: Potential Energy of Charged Particles
Activity 2: Potential Energy and Distance between Ions
Activity 3: Evaluating and Modifying a Model
At the atomic level, particles are most stable when energy is minimum. This happens for a sodium ion and a chloride ion when the ions are 276 pm apart (lowest point in the blue curve in Activity 3). Curves like this can be used to describe attractive forces between atoms, molecules, or ions. Particles attract each other so their potential energy decreases as they get closer, but eventually there are repulsive forces that prevent them from being in the same place at the same time. The balance of these forces results in a curve with a minimum at some distance of separation. The depth of the minimum indicates how strongly the particles attract.
D 1.6 Structure, Energy, and States of Matter
The idea that atomic-scale particles attract one another and adopt a low-energy arrangement unless energy is supplied from an external source can be applied to changes from solid to liquid to gas.
The kinetic-molecular theory states that atomic-level particles are in constant, random motion. How fast the particles move depends on temperature. As temperature increases the average speed of the particles increases; hence their kinetic energies also increase. The average of the energies of the particles is proportional to the absolute temperature (in units of kelvins). Near 0 K the particles have very little kinetic energy and adopt a structure with minimum potential energy.
Temperature and motion of particles affect whether a substance is a solid, liquid, or gas. View this simulation of the behavior of noble gas atoms in solids, liquids, and gases. Choose “States”, then choose “Neon” (upper right corner). Click on each of the boxes: “Solid”, “Liquid”, and “Gas” (or use “Heat” (below the container of molecules) to raise the temperature).
Based on the simulation, write in your notebook a description of the differences in position and movement of the molecules in solids, liquids, and gases.
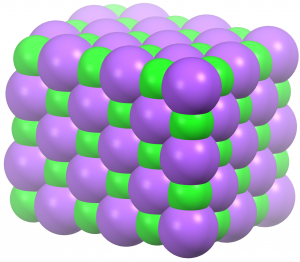
Let’s apply these ideas to sodium chloride, which is a solid at low temperature. Sodium ions and chloride ions are packed closely together in a regular pattern, as seen in the diagram at the right. This arrangement minimizes the potential energy by bringing oppositely charged particles closer together. A little above 0 K the ions are in motion: each vibrates a bit around its specific location, but no ion has sufficient kinetic energy to overcome the Coulomb’s-law attractions that hold it in place. Thus, no ion exchanges place with any other ion. This atomic-scale structure is consistent with macroscopic properties: a solid is rigid because the regular pattern of its structure does not change.
As temperature increases the average kinetic energy of the ions increases: the ions move more, vibrating farther from their average positions. On the macroscopic scale, the solid expands because each vibrating ion pushes against neighboring ions. Pushing neighboring ions away enlarges the space occupied by each ion and the crystal’s volume increases. Eventually, the vibrations are large enough that ions can move relative to each other and the regular arrangement becomes much more random: the solid melts. Ions are still close together but they can move past one another, so the liquid has no specific shape. The liquid is fluid and can be poured. For sodium chloride, because the potential energy is lowered a lot by Coulomb’s-Law attractions among the ions, the temperature required for melting is high: 1074 K (801 °C).
Further increase in temperature makes the motion of the atoms faster and at 1738 K (1465 °C) the liquid sodium chloride boils, forming a gas. The sodium ions and chloride ions move much further apart, which means they bump into each other much less often. The gas is fluid but expands to fill whatever volume is available and has much lower density than the liquid or solid.
D1.7 What’s Ahead?
Throughout this course you will build on the fundamental ideas of chemistry developed in this section. You will begin by studying the properties of atoms and how those properties vary depending on position in the periodic table. This will lead to how atoms lose or gain electrons to form ions and how atoms share electrons to form molecules, both of which are related to valence. Based on the properties of atomic-scale atoms and ions, you will be able to understand properties of metals and ionic compounds. The number and variety of molecules is very large so a lot of your study will involve how atoms bond to each other to form molecules and how molecular structures affect properties and reactivity of molecular substances. Then we will consider how fast chemical reactions go and to what extent products of those reactions can be formed from a given set of reactants. These ideas will then be applied to two important classes of reactions: acid-base reactions and oxidation-reduction reactions.
Bon voyage!
Activity 5: Wrap-up
Day 1 Pre-Class Podia Problem: Describe Chemistry
In most pre-class assignments we will include a Podia question that may require numerical, text, and/or image-based responses. Each pre-class Podia question is based on the pre-class material and working through the pre-class material will help you formulate your response. Consider the problem and write down/draw out your solution in your class notebook.
Two days before the next whole-class session, the pre-class Podia question will become visible in Podia (within the “Whole Class” section), where you can submit your solution. [For example, the Podia question in a Wednesday pre-class assignment will open by Monday evening.]
When you come to the next whole-class session, the class leader will choose one or more student-submitted solutions and explain why they are (or are not) effective scientific statements. These questions are designed to hone your skills so that you can solve mastery problems you will encounter on exams.
Click on the relevant open Podia question and submit your answer there. Your submission to instructor-opened questions will be anonymous to your classmates, and you can only view your own submission. (If you make a separate post, you will not be anonymous.)
You may find Podia sign-in instructions here.
Your first pre-class Podia question is designed to help you learn how Podia works. Write a short sentence about chemistry. Accompany your sentence with an image (sketched by you) that you think is a good example of chemistry. See whether your class leader selects your submission as a good one to show (anonymously) to the class.