Unit Two
Day 12: Intermolecular Forces; Functional Groups
Applying Core Ideas: Comparing Propane and Dimethyl Ether
D12.1 Intermolecular Forces: Dipole-Dipole Attractions
The additional IMF alluded to in the Applying Core Ideas box is called dipole-dipole attraction, attractive electrostatic forces between polar molecules. The attractive force arises when the positive end of one molecular dipole interacts with the negative end of another molecular dipole (Figure 1).
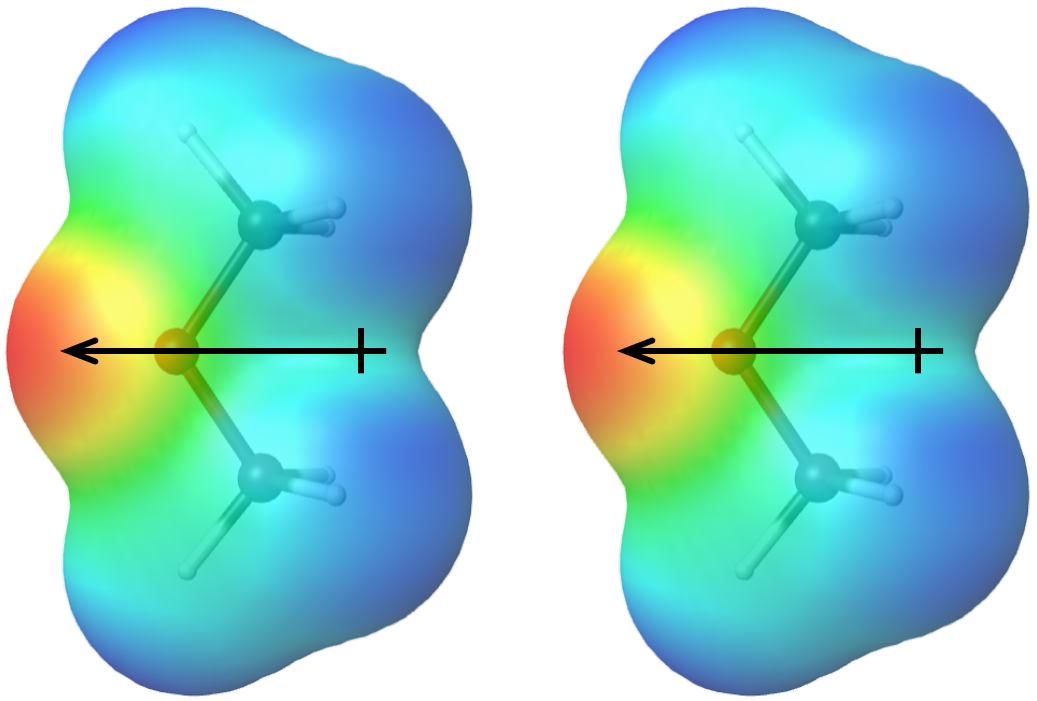
The more polar a molecule is (that is, the larger its molecular dipole moment is), the stronger the dipole-dipole attractions are between molecules of that substance. Molecular polarity depends both on the sizes of the bond dipoles (that is, on electronegativity differences between pairs of bonded atoms) and the shape of the molecule. Physical properties of a substance are influenced by all IMFs between molecules of the substance, so it is important to consider both LDFs and dipole-dipole attractions when predicting properties such as boiling points.
How do we know whether a molecule has a dipole moment? In Section D9.2 we described polar covalent bonds—bonds in which there is an unequal distribution of electron density on two bonded atoms and hence a bond dipole moment. The sum of all bond dipole moments in a molecule gives a molecular dipole moment. Molecules that have a molecular dipole moment are called polar molecules; molecules that have a zero (or near zero) molecular dipole moment are called nonpolar molecules.
To predict whether a molecule is polar, first determine whether there are polar bonds by comparing electronegativities of each pair of bonded atoms. If electronegativity differences are small or zero, there are no polar bonds and the molecule must be nonpolar. If there are polar bonds, the molecule might be polar, but it is also possible that the bond dipoles might cancel. For example, both carbon dioxide (CO2) and sulfur dioxide (SO2) have polar bonds, but only SO2 is polar. In CO2, the central carbon has two σ bonds, it is sp hybridized, and therefore the molecule is linear. The bond dipoles are large (ΔEN = 3.5 − 2.6 = 0.9) and equal in magnitude , but they are pointing in exact opposite directions. This results in a molecular dipole moment of zero. In SO2, where the central S atom has two σ bonds and one lone pair, the S atom is sp2 hybridized and the molecule is bent. Thus, the bond dipoles are also large (ΔEN = 3.5 − 2.6 = 0.9), but they are at an angle and the resultant molecular dipole is not zero.
Bond dipoles behave as vectors, so if you are familiar with vector addition you can predict when bond dipoles cancel and when they do not. Another way to predict is this: molecules with all terminal atoms the same and no lone pairs on the central atom are nonpolar because of cancellation of bond dipoles. (In the case of a molecule with an odd number of electrons, a single electron on the central atom counts as a lone pair.) For multicentered molecules, predicting molecular dipoles is trickier. Generally, if atoms have similar electronegativities, then bond dipoles are weak and the molecular dipole moment is small. For example, because C and H have similar electronegativity, C-H bonds have small bond polarity, and hydrocarbon molecules are nonpolar. The dipole moment of propane, for example, is less than 0.1 D—essentially negligible.
Exercise 1: Predicting Molecular Polarity
Exercise 2: Predicting Boiling Points
D12.2 Functional Groups
There are more than twenty million known organic compounds, so it would be impossible to memorize chemical properties for each one. Fortunately, we can make use of functional groups to deduce the likely chemical and physical properties of a molecule. A functional group is an atom or group of atoms that has similar chemical properties whenever it is present in a molecule. Even if other parts of a molecule are quite different, a specific functional group usually reacts the same way.
Because C–C and C–H bonds are strong, alkanes are unreactive at room temperature; they are used primarily as fuels (Section D7.2). The alkane parts of molecules usually don’t participate in reactions and are not defined as functional groups. An alkyl group is a portion of an alkane molecule bonded to something else. Examples of alkyl groups are -CH3 (methyl), -CH3CH2 (ethyl), and -CH(CH3)2 (2-propyl). (See alkane nomenclature for more examples.) We often use R (for the Rest of the molecule) to designate any alkyl group (or sometimes another type of group) in a molecule. When there are two or more different alkyl groups, we use R, R’, R”, etc. For example, R and R’, are trans to each other in the alkene structure below:
When a molecule is drawn using R or R’ for alkyl groups, greater focus is put on a specific functional group, in this case, the alkene C=C bond.
Sections D7.3 and D7.4 described the functional groups in alkenes and alkynes. The aromatic functional group was discussed in Section D9.6. The next few sections consider functional groups that contain heteroatoms: atoms other than carbon and hydrogen. Each of these functional groups has its own specific reactivity. Each functional group can also affect the types of intermolecular forces, giving rise to differing physical properties.
D12.3 Aldehydes and Ketones
An aldehyde or a ketone contains a carbonyl group, a carbon atom double bonded to an oxygen atom. The carbon atom in a carbonyl group is called the carbonyl carbon. In an aldehyde functional group, the carbonyl carbon is also bonded to a hydrogen atom. Hence, an aldehyde group can only bond to one R group (another carbon atom or a H atom), and the aldehyde group is always at the end of a chain of carbon atoms (click on the image below for a 3D model.)
A ketone functional group consists solely of the carbonyl group. It bonds to two R groups, which may be the same or different, and is found partway along of a chain of carbon atoms. (click on the image below for a 3D model.)
The reactivity of both aldehydes and ketones are directly related to the reactivity of the carbonyl group.
Formaldehyde is a colorless gas with a pungent and irritating odor. It is sold in an aqueous solution called formalin, which contains about 37% formaldehyde by mass.
Formaldehyde causes coagulation of proteins, so it kills bacteria (and any other living organism) and stops many of the biological processes that cause tissue to decay. Thus, formaldehyde is used for preserving tissue specimens and embalming bodies. It is also used to sterilize soil or other materials. Formaldehyde is used in the manufacture of Bakelite, a hard plastic having high chemical and electrical resistance.
Dimethyl ketone, CH3COCH3, commonly called acetone, is the simplest ketone. It is a colorless liquid that can be made commercially by fermenting corn or molasses. Among its many uses are as a solvent for lacquer (including fingernail polish), cellulose acetate, cellulose nitrate, acetylene, plastics, and varnishes; as a paint and varnish remover; and as a solvent in the manufacture of pharmaceuticals and chemicals.
Activity 1: Ketone Hybridization and Local Bond Geometry
D12.4 Ethers
An ether functional group contains the group –O–, which bonds to two different R groups and is found in the middle of a molecule.
Diethyl ether, the most widely used compound of this class, is a colorless, volatile liquid that is highly flammable. It was first used in 1846 as an anesthetic, but better anesthetics have now largely taken its place. Diethyl ether and other ethers are now used primarily as solvents for gums, fats, waxes, and resins. Methyl tert-butyl ether (abbreviated MTBE) is used as an additive for gasoline. MTBE belongs to a group of chemicals known as oxygenates due to their capacity to increase the oxygen content of gasoline.
Activity 2: Ether Hybridization and Local Bond Geometry
D12.5 Esters
An ester functional group contains a carbonyl group with a second oxygen atom single bonded to the carbonyl carbon and also single bonded to another carbon atom. A general ester structure has an R group bonded to the carbonyl carbon atom and another R group bonded to the second oxygen. It is a functional group that is found in the middle of a molecule. (Click on the image below for a 3D model.)
The ester functional group’s carbon atom is sp2 hybridized with a trigonal planar local geometry. Its carbonyl oxygen is sp hybridized, and one of its unhybridized 2p AOs forms the π bond with the carbon’s unhybridized 2p AO. This oxygen also has two lone pairs: one occupies a sp hybrid orbital; the other occupies a 2p AO that is perpendicular to the π bond. The second oxygen (non-carbonyl oxygen) is sp2 hybridized and has a bent local geometry. It also has two lone pairs, one in a sp2 hybrid orbital, the other in the unhybridized 2p AO. See Figure 2 below.
As shown in Figure 2, the 2p lone pair on the non-carbonyl O is aligned parallel to the p orbitals that form the π bond. This leads to some delocalization of the lone pair electron densities, which can be expressed by resonance structures:
While the resonance structure on the right makes only a minor contribution to the description of the ester molecule, that structure is important in understanding the ester’s chemical and physical properties. For example, the -COO- ester group is planar, and the non-carbonyl C-O bond is not as freely rotatable as a typical single bond. Moreover, an ester’s reactivity is quite different from that of a ketone or an ether, and hence an ester is a distinct functional group.
Activity 3: Ester Hybridization and Local Bond Geometry
The odors of ripe bananas and many other fruits are due to the presence of esters.
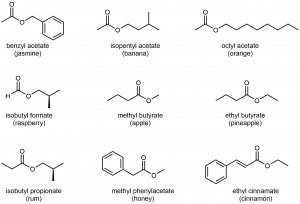
Day 12 Pre-class Podia Problem: Predicting Boiling Points
The observed boiling points for CCl4 and CHCl3 are:
CCl4 : 77 °C CHCl3 : 61 °C
Use your knowledge of intermolecular forces to write an explanation for why CCl4 has a higher boiling point.
Two days before the next whole-class session, this Podia question will become live on Podia, where you can submit your answer.