Module 21: Visual System
Learning Objectives:
By the end of this class, students will be able to:
- Describe the bones of the skull that make up the orbit.
- List and explain the function of the extrinsic muscles of the eye.
- Describe the anatomy of the eye, including the layers, and the function of all relevant structures.
- Describe vision receptors in the retina.
- Describe the blood supply to the retina.
- Explain the primary visual pathway and accessory pathways of the visual system.
- Describe the regions of the brain involved in vision.
Terms to Know
Eye
*Covered only in lecture, not in this text |
Extrinsic Eye Muscles
Accessory Structures
Visual Pathways
|
Accessory Structures of the Eye
This content will be covered in lecture.
The eyes are located within either orbit in the skull. The bony orbits surround the eyeballs, protecting them and anchoring the soft tissues of the eye. Several bones contribute to the orbit, including:
- Roof: Frontal bone
- Lateral wall: Zygomatic bone
- Floor: Maxillary bone and palatine bone
- Medial wall: Lacrimal bone and ethmoid bone
- Posterior wall: Sphenoid bone
The eyelids, with lashes at their leading edges, help to protect the eye from abrasions by blocking particles that may land on the surface of the eye. The space between the upper and lower eyelids is called the palpebral fissure. The medial and lateral angles are where the upper and lower eyelids meet on the medial and lateral aspects of the orbit. The eyelids are composed of very thin layers of skin and tissue. To ensure they maintain their shape as they move over the anterior surface of the eye, the eyelids each contain a layer of connective tissue called a tarsal plate. The orbicularis oculi muscle also inserts onto the tarsal plates. The eyelids also contain modified sebaceous glands, called tarsal glands, that lubricate the surface of the eye, prevent tear overflow, and prevent the eyelids from sticking together.
The inner surface of each lid is a thin membrane known as the palpebral conjunctiva. The conjunctiva extends over the white areas of the eye (the sclera), connecting the eyelids to the eyeball. The portion of the conjunctiva that covers the anterior eye is called the bulbar conjunctiva. Collectively, the conjunctiva is a transparent mucous membrane made of primarily stratified squamous epithelium.
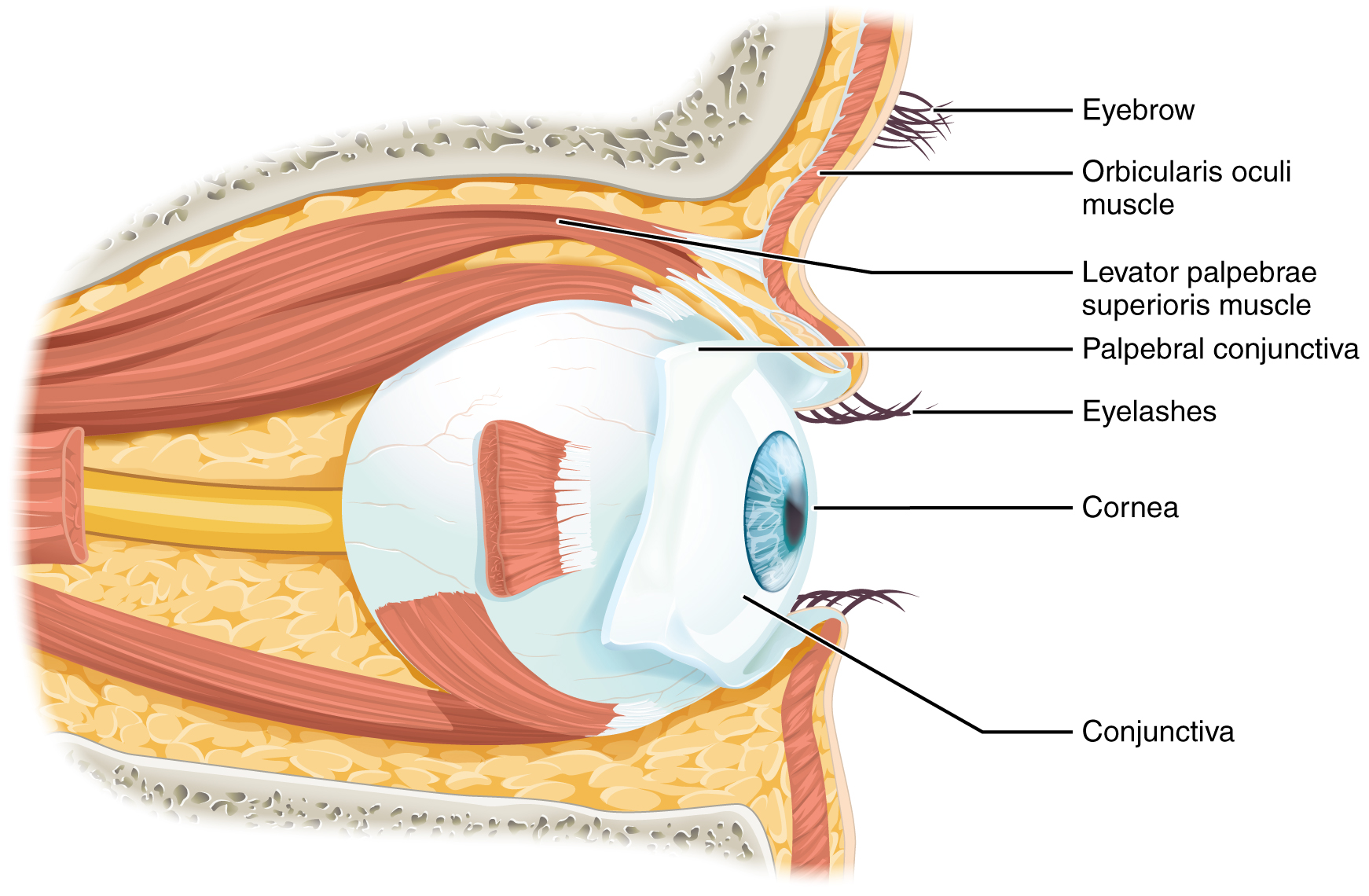
Lacrimal Apparatus
Tears, or lacrimal fluid, are produced by the lacrimal gland, located in the superior lateral aspect of the orbit. See the lecture slides for the functions of lacrimal fluid and the detailed pathway that lacrimal fluid takes from production to draining into the nasolacrimal duct.
Muscles That Move the Eye
This content will be covered in lecture.
Movement of the eye within the orbit is accomplished by the contraction of six extraocular muscles that originate from the bones of the orbit and insert into the sclera, or white surface of the eye. Four of the muscles around the eye are named for their locations: the superior rectus, medial rectus, inferior rectus, and lateral rectus. They originate in the posterior aspect of the orbit at a common tendinous ring. When each of these muscles contract the eye moves toward the contracting muscle. (See the lecture slides).
The superior oblique originates at the posterior orbit, near the origin of the four rectus muscles. However, the tendon of the oblique muscles threads through a pulley-like piece of cartilage known as the trochlea. The tendon inserts obliquely into the superior surface of the eye. The angle of the tendon through the trochlea means that contraction of the superior oblique depresses (rotates inferiorly) and abducts (rotates laterally) the eye. The inferior oblique muscle originates from the floor of the orbit and inserts into the inferolateral surface of the eye. When it contracts, it elevates (rotating superiorly) and abducts the eye.
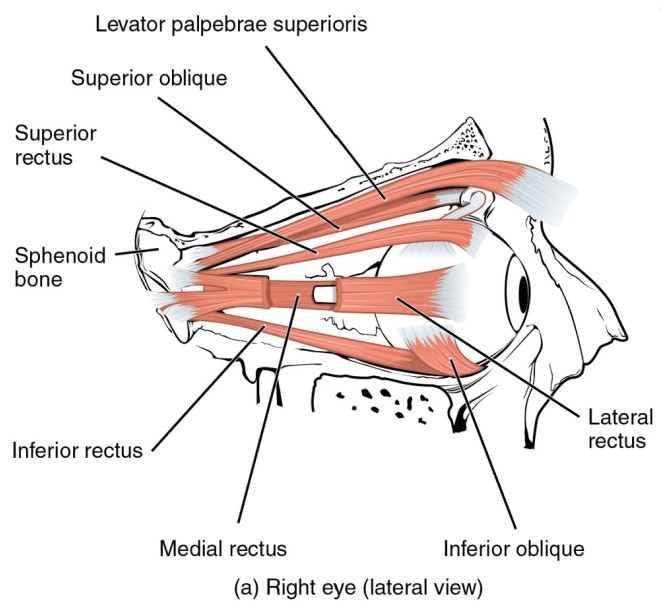
The extraocular muscles are innervated by three cranial nerves. The lateral rectus, which causes abduction of the eye, is the only muscle innervated by the abducens nerve. The superior oblique is the only muscle innervated by the trochlear nerve. The other four extraocular muscles are innervated by the oculomotor nerve.
Anatomy of the Eye
This content will be covered in the assignment, and some of it will be reviewed and built upon in lecture.
The eye is divided into two cavities: the anterior cavity and the posterior cavity. The anterior cavity is the space between the cornea and lens. It is divided into an anterior and posterior chamber by the iris. It is filled with a watery fluid called the aqueous humor. The posterior cavity is the space behind the lens that extends to the retina. The posterior cavity is filled with a more viscous fluid called the vitreous humor.
The eye itself is a hollow sphere composed of three layers of tissue:
Fibrous Tunic
The outermost layer of the eye is the fibrous tunic, which includes the sclera and cornea.
The sclera is the white portion of the eye. It accounts for the majority of the surface of the eye, most of which is not visible. It is the site of attachment for the extraocular muscles.
Continuous with the sclera, the transparent cornea is the most anterior portion of the eye. It is clear, and it allows light to enter the eye. It is covered by stratified squamous epithelium. The cornea is avascular, meaning it does not have its own blood supply. Instead, it receives its oxygen and nutrients from the lacrimal fluid anterior to it and aqueous humor posterior to it. Though it does not have blood vessels supplying it, the cornea is highly innervated. It has abundant free nerve endings, which makes it very sensitive to pain.
Vascular Tunic
The middle layer of the eye is the vascular tunic, which includes the choroid, ciliary body, and iris.
The choroid is a layer of highly vascularized connective tissue. It contains an extensive capillary network that delivers nutrients to the retina. It’s just deep to the sclera and posterior to the other structures of this layer.
The ciliary body consists of the ciliary muscles, a muscular ring projecting into the eye, and the ciliary processes. The ciliary processes are attached to the lens by suspensory ligaments, or zonule fibers, which suspend the lens in place and pull on the lens when tension is placed on them by the ciliary muscle. Together, these structures adjust the shape of the lens, allowing it to focus light on the back of the eye.
Superficial to the ciliary body, and visible in the anterior eye, is the iris. It contains pigmented cells that give us our eye color. The iris contains intrinsic muscles of the eye, or the smooth muscles located completely within the eye that open or close the pupil, which is the hole at the center of the eye that allows light to enter. The sphincter pupillae functions to reduce the diameter of the pupil, while the dilator pupillae functions to increase the diameter of the pupil. These muscles are under autonomic control.
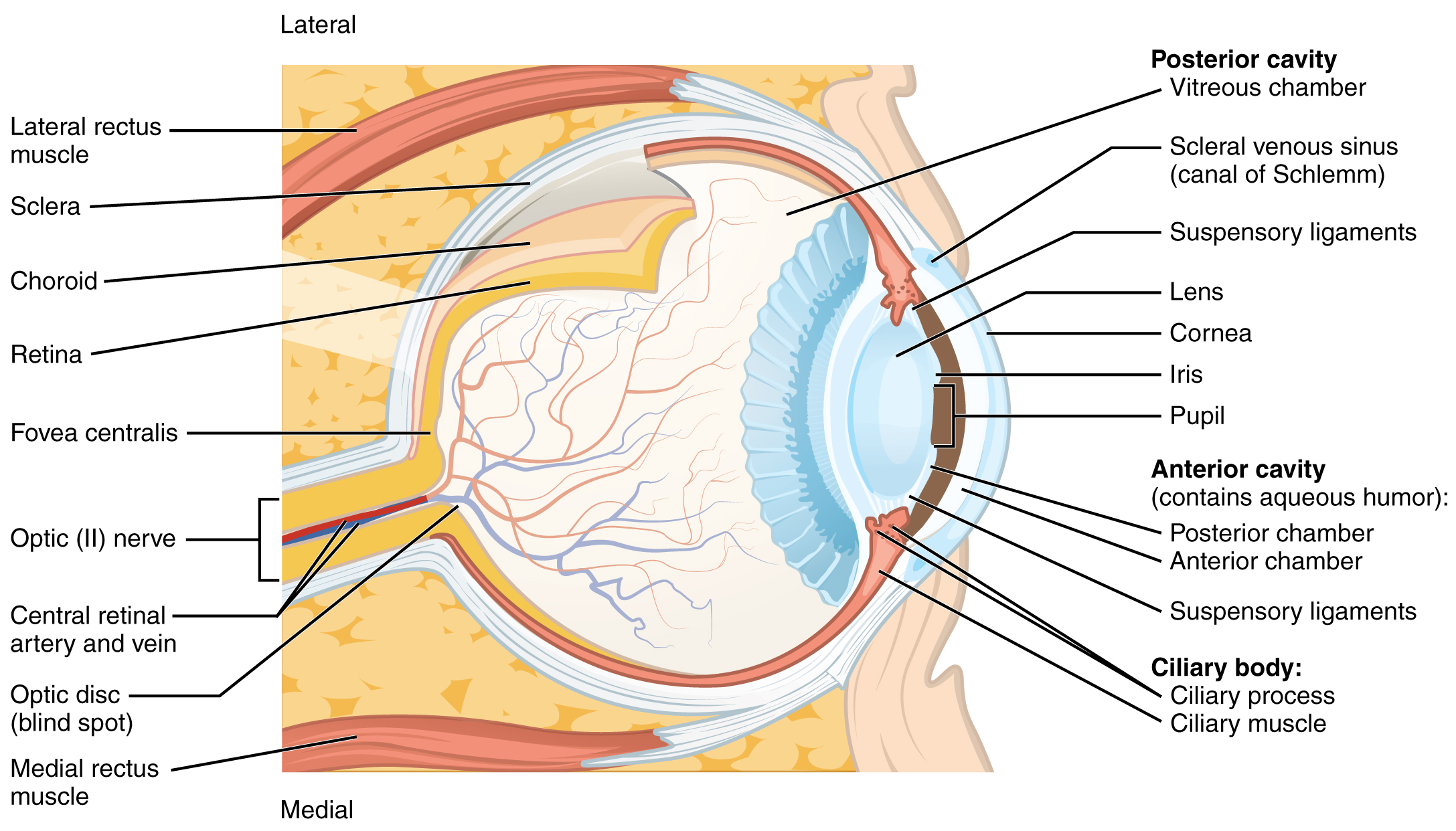
Retina
The innermost layer of the eye is the retina, which contains the nervous tissue responsible for photoreception and the initial processing of visual stimuli. The retina has two sublayers. The pigmented layer absorbs light and, in response, undergoes important biochemical interactions that trigger activity in the photoreceptors. The neural layer contains the photoreceptors as well as supporting cells and neurons that perform the first steps in visual processing and integrating visual information.
The two types of photoreceptor cells, rods and cones, are stimulated by different types of light.
- Rods: responsible for vision in dim light. They are not responsible for color vision. Instead they are sensitive to very low levels of light, allowing us to see in relatively dark settings. However, they have low spatial acuity, meaning they are not capable of sharp, high-definition vision. Rods are more highly concentrated towards the peripheral aspect of the retina, and fewer are located in central portions of the retina.
- Cones: responsible for color vision and high-acuity vision, allowing us to see sharper, clearer color images. They do not function well in dim lighting. Cones are most concentrated in the central portion of the retina.
At the center of the retina is a small area called the macula, which contains a high density of cones. At the center of the macula is the fovea, which is almost entirely made of cones. Therefore, visual acuity, or the sharpness of vision, is greatest at the fovea (fovea centralis). As one moves in either direction from this central point of the retina, visual acuity drops significantly. The images in your peripheral vision are focused by the peripheral retina, which contains a higher density of rods. As a result, a large part of the neural function of the eyes is concerned with moving the eyes and head so that important visual stimuli are centered on the fovea.
The photoreceptors synapse with bipolar cells. This is one of the few places where we have bipolar cells in the body (we also have them in the olfactory pathway). The short bipolar cells then synapse with the much longer retinal ganglion cells. The axons of retinal ganglion cells, which lie at the innermost layer of the retina, collect at the optic disc and leave the eye as the optic nerve. Because these axons converge and pass through the retina, there are no photoreceptors at the optic disc, where the optic nerve begins. This creates a “blind spot” in the retina, and a corresponding blind spot in our visual field.
The pigmented layer is the most superficial layer of the retina, closer to the surface of the eye. However, light is entering the retina from the internal aspect of the eye. As a result, the light has to pass through the entire retina, past the retinal ganglion cells, bipolar cells, and pigmented cells, before it can be absorbed in the pigmented layer.
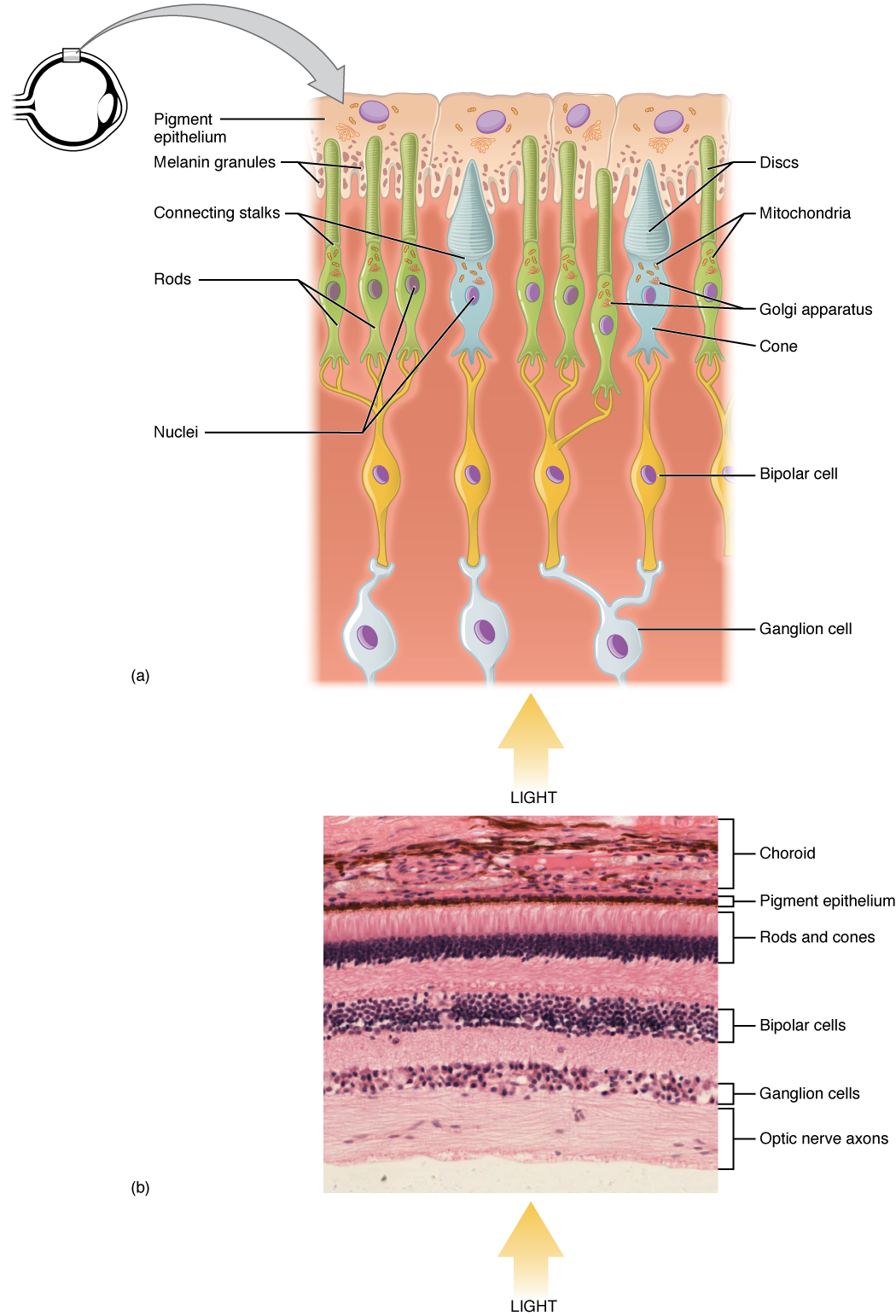
Visual Pathways
This information will be covered in lecture.
Each retina can be divided into a nasal retina, the medial half of the retina closest to the nose, and a temporal retina, the lateral half of the retina near the temporal bone. The visual field is everything that we can see in a given moment. Because of the posterior curve of the retina, the light information that reaches the temporal aspect of the retina has come from the medial aspect of primarily the opposite side of the visual field. The light information that reaches the nasal retina has come from the peripheral aspect of the visual field (lateral). As a result, one eye can see aspects of both the right and left halves of the visual field.
The curve of the retina also causes the visual field to be processed essentially upside down on the retina. The superior portion of the retina is receiving light information from the bottom half of the visual field, while the inferior portion of the retina is receiving light information from the superior portion of the visual field.
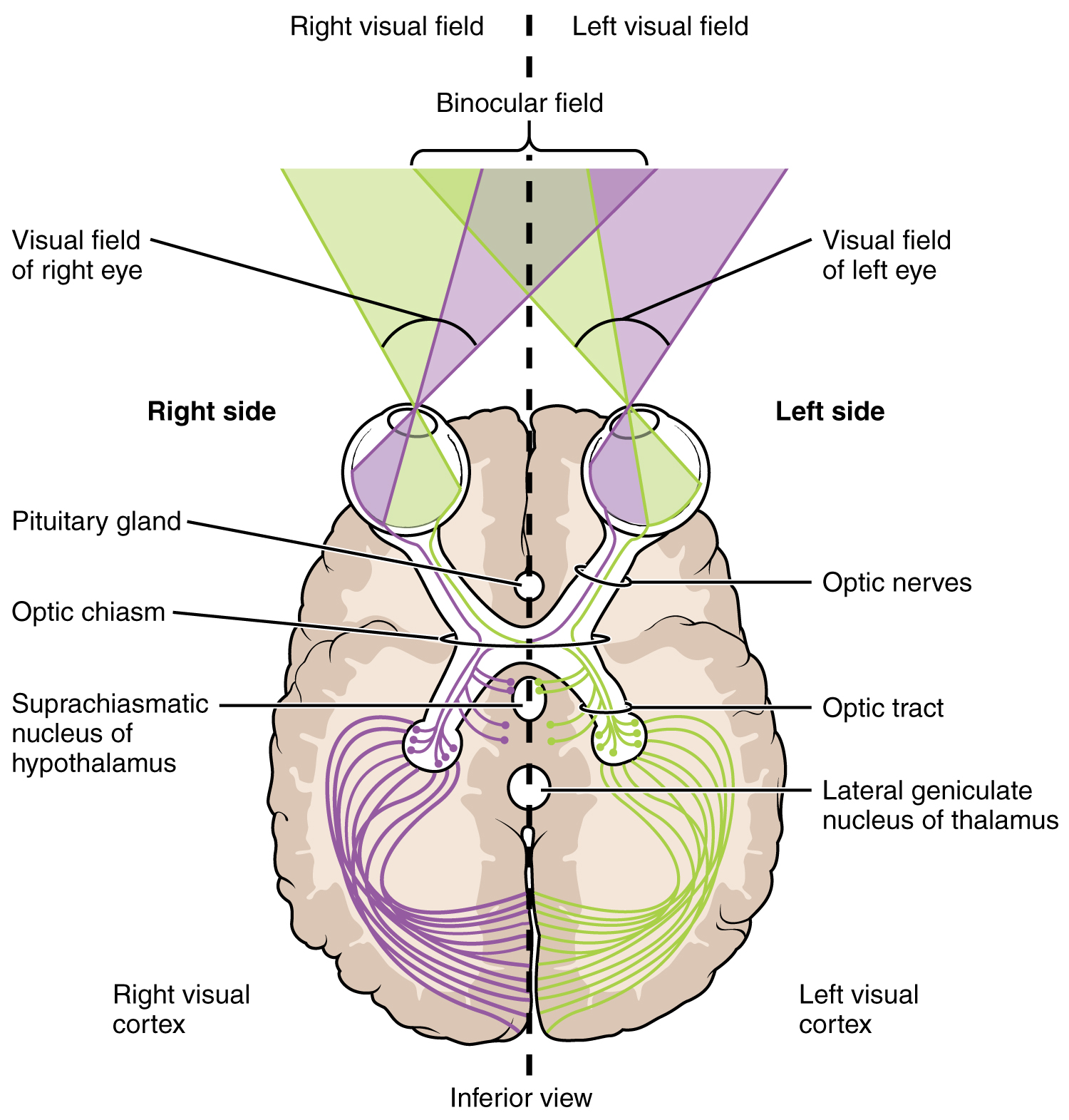
Axons of retinal ganglion cells of the same eye merge together and leave the eye as the optic nerve (cranial nerve II). The axons originating from the nasal retina decussate at the optic chiasm. However, the axons originating from the temporal retina do not decussate. This allows one entire half of the visual field to be processed by the opposite half of the brain. For example, axons from the nasal portion of the right eye, carrying information from the right periphery, decussate at the optic chiasm and merge with axons from the temporal portion of the left eye, which carry information from the central portion of the right visual field. As a result, the entire right visual field is now traveling and being processed in the left side of the brain.
Extending from the optic chiasm, the axons of the visual system are referred to as the optic tract. However, there has not been a synapse yet. The axons of the optic tract are still the same axons of the retinal ganglion cells that originated in the retina.
Ninety percent of the optic tract fibers travel to the lateral geniculate nucleus of the thalamus. Axons from this nucleus then project to the primary visual cortex of the cerebrum, located in the occipital lobe, via the optic radiations.
The other 10% of axons in the optic tract travel to three locations:
- Superior colliculus: Located in the midbrain, this nucleus is involved in visual reflexes. When we see something in our periphery, this nucleus is involved in a pathway that causes us to turn our head so that we can focus the visual information on the macula, the portion of the retina with the highest-acuity vision, and determine what it is.
- Suprachiasmatic nucleus: Located in the hypothalamus, this nucleus senses the presence of absence of light and plays a role in our sleep-wake cycle. If light is not present (nighttime), it will send information to the pineal gland, signaling it to secrete melatonin, which makes us sleepy. If light is present (daytime), it will suppress the pineal gland and prevent it from secreting melatonin.
- Edinger–Westphal nucleus: Located in the midbrain, this nucleus is involved in pupillary reflex and accommodation of the lens. Axons originating in this nucleus travel with the fibers in the oculomotor nerve (cranial nerve III) to reach the ciliary ganglion. The postganglionic parasympathetic fibers then project to the smooth muscle of the iris to constrict the pupil in the presence of brighter light and to the ciliary muscle to adjust the shape of the lens for near vision.
Cortical Processing of Vision
This information will be covered in lecture.
The primary visual cortex of the occipital lobe is the first cortical area that processes visual information. The entire visual field is represented on the primary visual cortex. Information from the foveal region of the retina is processed in the center of the primary visual cortex. Information from the peripheral regions of the retina are correspondingly processed toward the edges of the visual cortex. Similar to the exaggerations in the sensory homunculus of the somatosensory cortex, the foveal-processing area of the visual cortex is disproportionately larger than the areas processing peripheral vision.
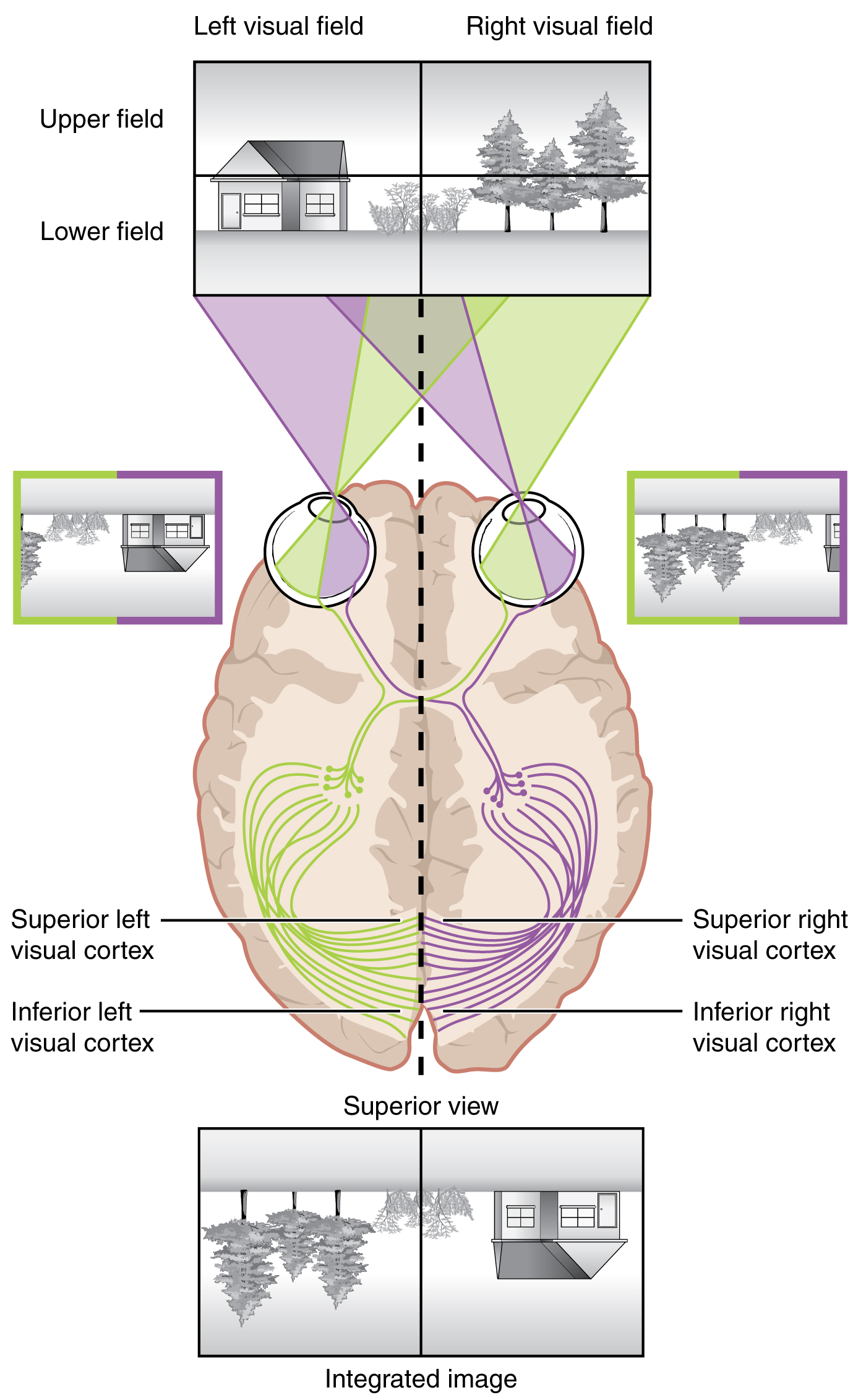
There are several cortical regions surrounding the primary visual cortex that are also dedicated to processing visual information. They are referred to as the visual association cortices. Most are located in the occipital lobe, as the entire occipital lobe is dedicated to vision. The two main regions that surround the primary cortex are usually referred to as areas V2 and V3 (the primary visual cortex is area V1), and there are additional “Vs” (V4, V5, V6, etc.). Each “V” processes the entire visual field but adds something additional to what we see, developing more complex visual perceptions by adding color and motion information, for example.
The information processed in the visual association areas is then sent to regions of the temporal and parietal lobes. Visual processing has two separate streams of processing: one into the temporal lobe and one into the parietal lobe. These are the ventral and dorsal streams, respectively. The ventral stream identifies visual stimuli and their significance and is sometimes referred to as the “what” stream. Because the ventral stream uses temporal lobe structures, it begins to interact with the non-visual cortex and may be important in integrating visual stimuli with memories. The dorsal stream locates objects in space and helps in guiding movements of the body in response to visual inputs. The dorsal stream is sometimes referred to as the “where” stream, as it involves parietal lobe structures involved in visuospatial processing, helping us understand where the source of visual stimuli (what we are seeing) is located in space.
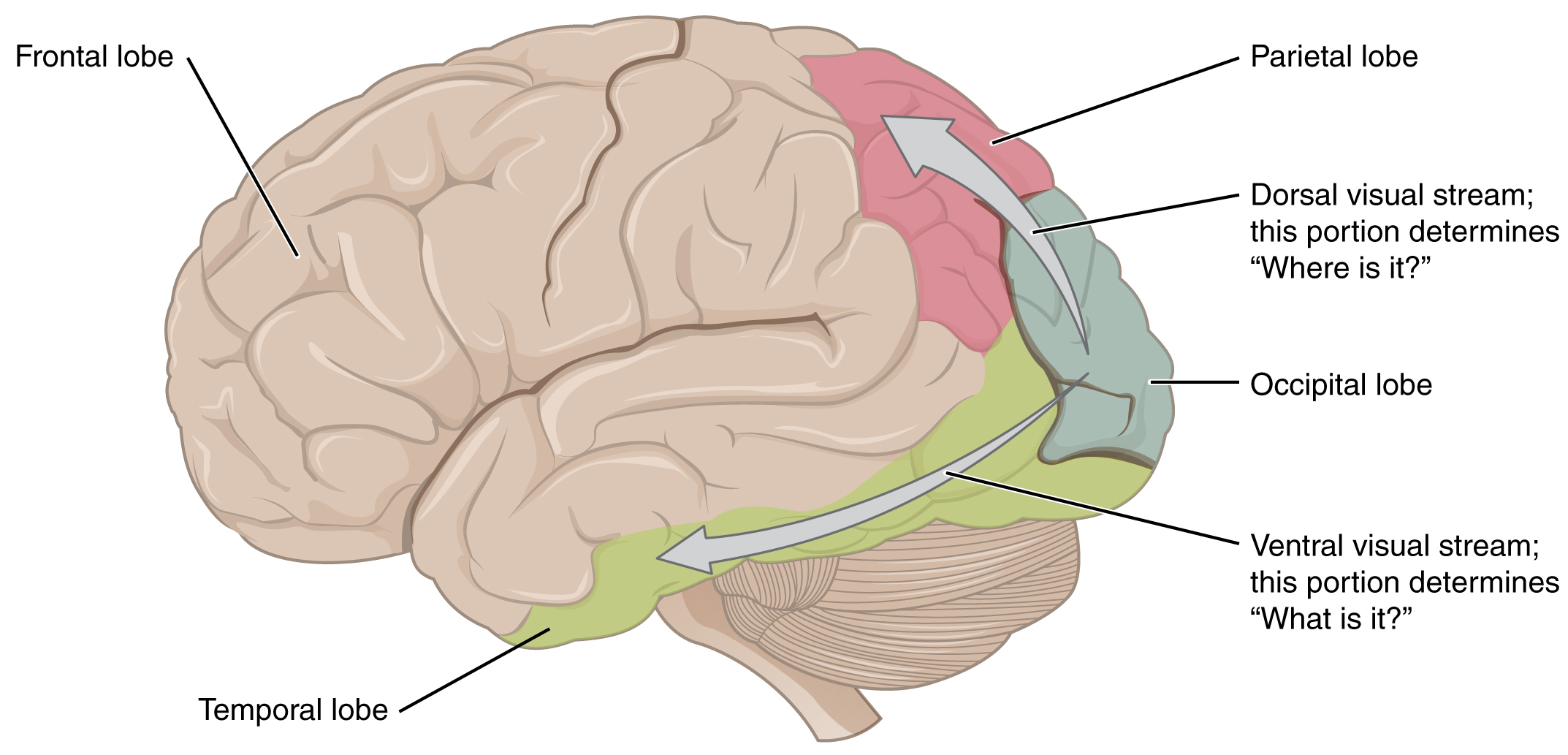