Module 12: Respiratory System
Learning Objectives:
By the end of this module, students will be able to:
- List the components of the conducting and respiratory portions of the respiratory system.
- Describe the functions of the respiratory system.
- Describe the structure and functions of the trachea, bronchi, and lungs.
- Identify the blood supply to lung tissue.
- Explain the mechanics of breathing.
- Divide the mediastinum into the sub-divisions.
- List the components of the mediastinum.
Terms to Know
General
Trachea
Bronchi and Bronchioles
|
Lungs
Alveoli
Breathing
|
*This content is introduced in the pre-lecture assignment and will be elaborated on in lecture.
The major organs of the respiratory system function primarily to provide oxygen to body tissues for cellular respiration, remove the waste product carbon dioxide, and help to maintain acid-base balance. Portions of the respiratory system are also used for non-vital functions, such as sensing odors, speech production, and straining, such as during childbirth or coughing.
Functionally, the respiratory system can be divided into a conducting zone and a respiratory zone. The conducting zone of the respiratory system includes the organs and structures not directly involved in gas exchange. The gas exchange occurs in the respiratory zone.
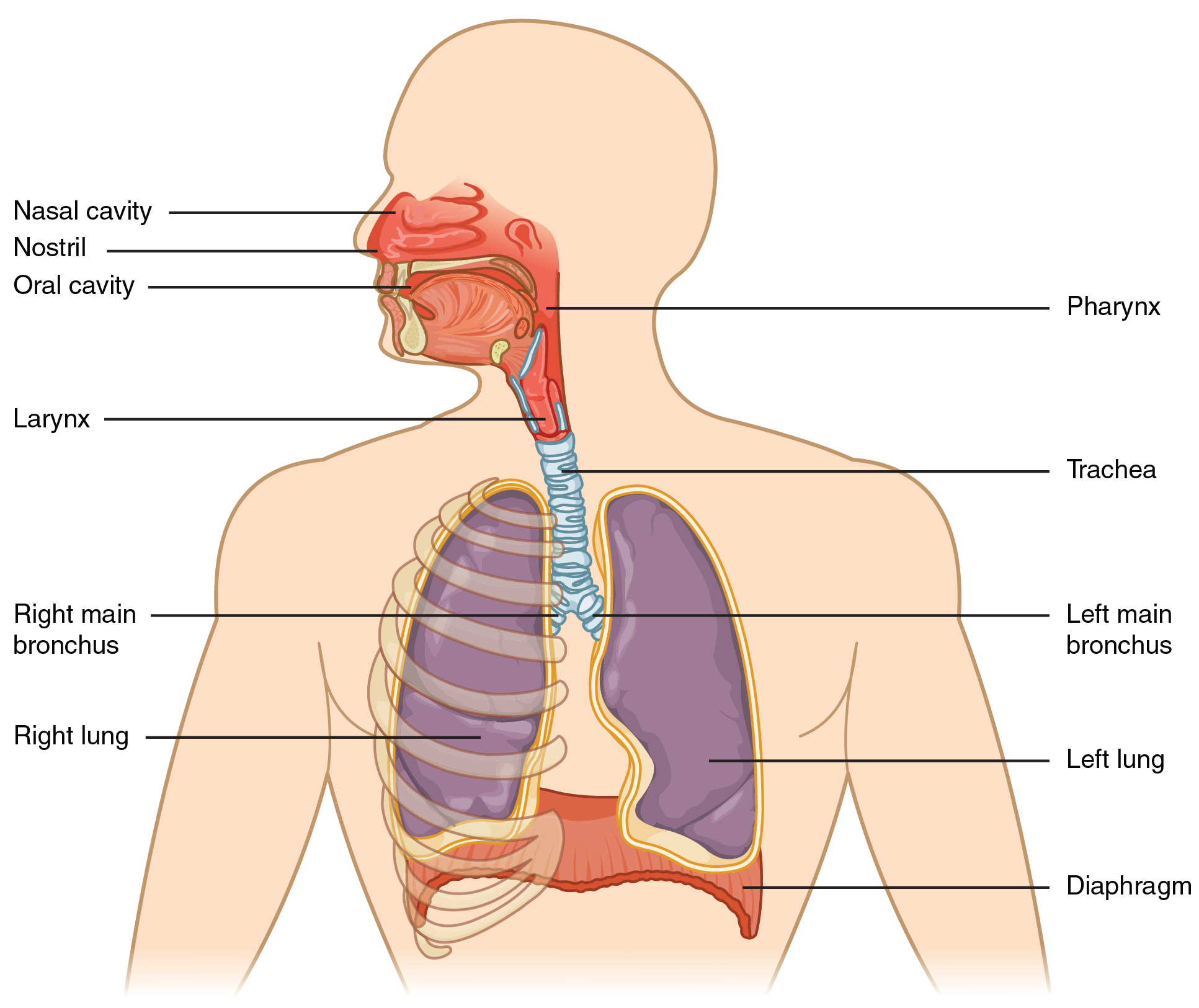
Conducting Zone
The major functions of the conducting zone are to provide a route for incoming and outgoing air, remove debris and pathogens from the incoming air, and warm and humidify the incoming air. Several structures within the conducting zone perform other functions as well. The epithelium of the nasal passages, for example, is essential to sensing odors, and the bronchial epithelium that lines the lungs can metabolize some airborne carcinogens.
Several bones that help form the walls of the nasal cavity have air-containing spaces called the paranasal sinuses, which serve to warm and humidify incoming air. Sinuses are lined with a mucosa. Each paranasal sinus is named for its associated bone: frontal sinus, maxillary sinus, sphenoidal sinus, and ethmoidal sinus. The sinuses produce mucus and lighten the weight of the skull.
The nares and anterior portion of the nasal cavities are lined with mucous membranes, containing sebaceous glands and hair follicles that serve to prevent large debris, such as dirt, through the nasal cavity. An olfactory epithelium used to detect odors is found deeper in the nasal cavity.
The conchae, meatuses, and paranasal sinuses are lined by respiratory epithelium composed of pseudostratified ciliated columnar epithelium. The epithelium contains goblet cells, one of the specialized columnar epithelial cells that produce mucus to trap debris. The cilia of the respiratory epithelium help remove the mucus and debris from the nasal cavity with a constant beating motion, sweeping materials towards the throat to be swallowed. Interestingly, cold air slows the cilia movement, resulting in the accumulation of mucus that may, in turn, lead to a runny nose during cold weather. This moist epithelium functions to warm and humidify incoming air. Capillaries located just beneath the nasal epithelium warm the air by convection. Serous and mucus-producing cells also secrete the lysozyme enzyme and proteins called defensins, which have antibacterial properties. Immune cells that patrol the connective tissue deep to the respiratory epithelium provide additional protection.
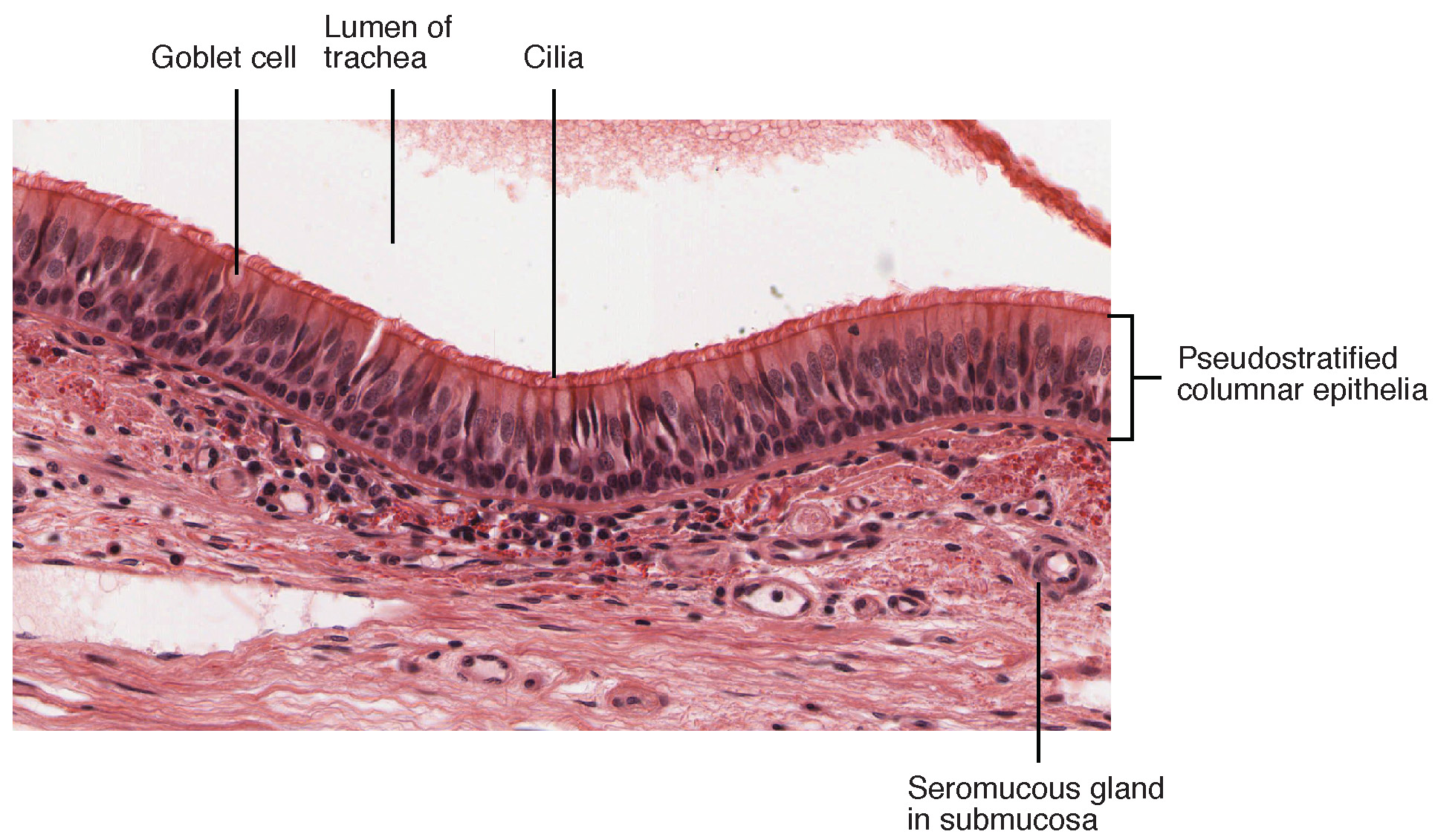
Trachea
The trachea (windpipe) extends from the larynx toward the lungs. The trachea is formed by 16 to 20 stacked, C-shaped hyaline cartilage pieces connected by dense connective tissue. The trachealis muscle and elastic connective tissue together form the fibroelastic membrane, a flexible membrane that closes the posterior surface of the trachea, connecting the C-shaped cartilages. The fibroelastic membrane allows the trachea to stretch and expand slightly during inhalation and exhalation, whereas the rings of cartilage provide structural support and prevent the trachea from collapsing. Also, the trachealis muscle can be contracted to force air through the trachea during exhalation. The trachea is lined with pseudostratified ciliated columnar epithelium, which is continuous with the larynx. The esophagus borders the trachea posteriorly.
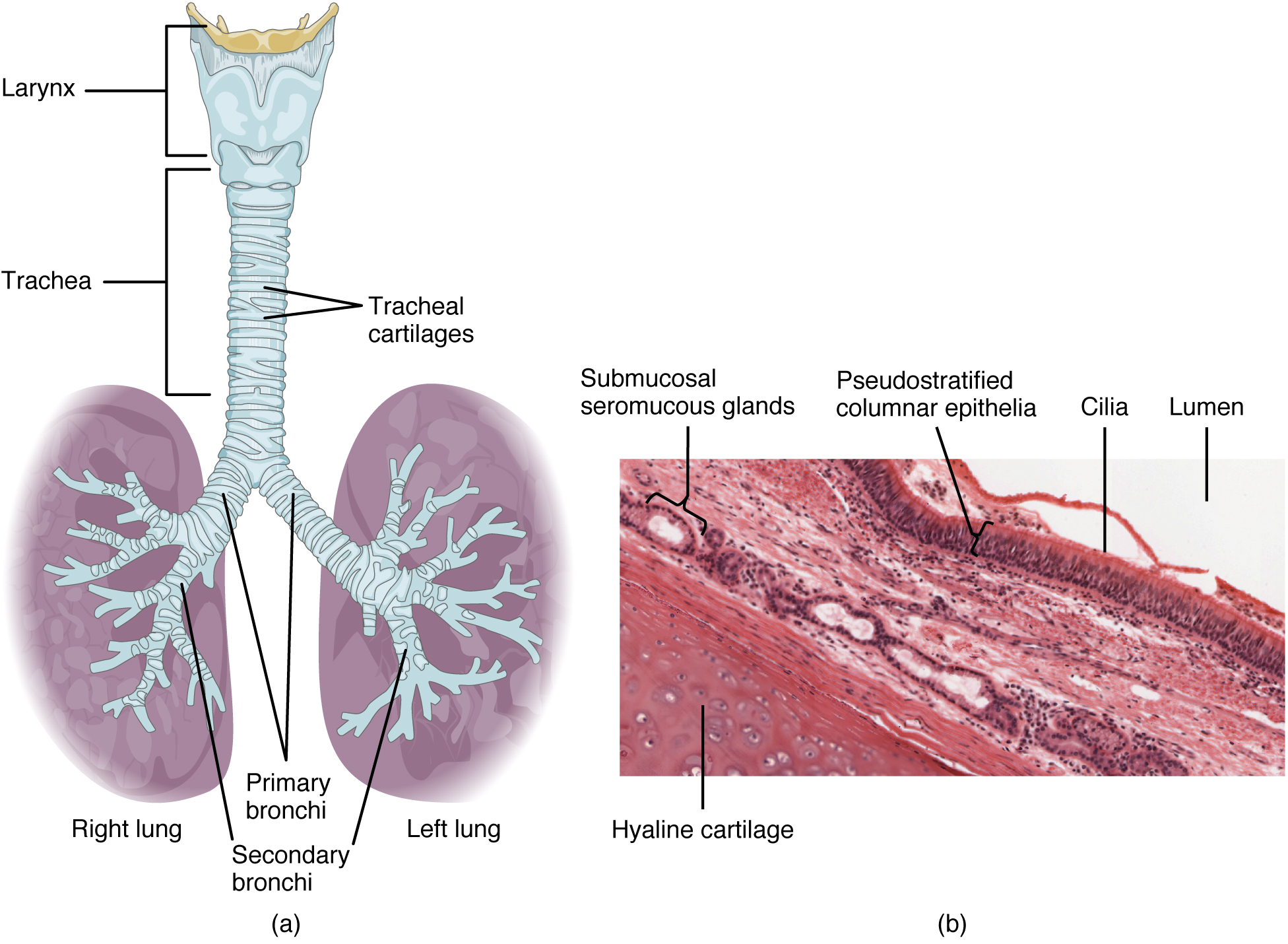
Bronchial Tree
The trachea branches into the right and left primary bronchi at the carina. These bronchi are also lined by pseudostratified ciliated columnar epithelium containing mucus-producing goblet cells. The carina is a raised structure containing specialized nervous tissue that induces violent coughing if a foreign body, such as food, is present. Rings of cartilage, similar to those of the trachea, support the structure of the bronchi and prevent their collapse. The primary bronchi enter the lungs at the hilum, a concave region where blood vessels, lymphatic vessels, and nerves also enter the lungs. The bronchi continue to branch into a bronchial tree. A bronchial tree (or respiratory tree) is the collective term used for these multiple-branched bronchi. Like other conducting zone structures, the main function of the bronchi is to provide a passageway for air to move into and out of each lung. Also, the mucous membrane traps debris and pathogens.
A bronchiole branches from the tertiary bronchi. Bronchioles, which are about 1 mm in diameter, further branch until they become the tiny terminal bronchioles, which lead to the structures of gas exchange. There are more than 1000 terminal bronchioles in each lung. The muscular walls of the bronchioles do not contain cartilage like those of the bronchi. This muscular wall can change the size of the tubing to increase or decrease airflow through the tube.
Respiratory Zone
In contrast to the conducting zone, the respiratory zone includes structures directly involved in gas exchange. The respiratory zone begins where the terminal bronchioles join a respiratory bronchiole, the smallest type of bronchiole, leading to an alveolar duct, opening into a cluster of alveoli.
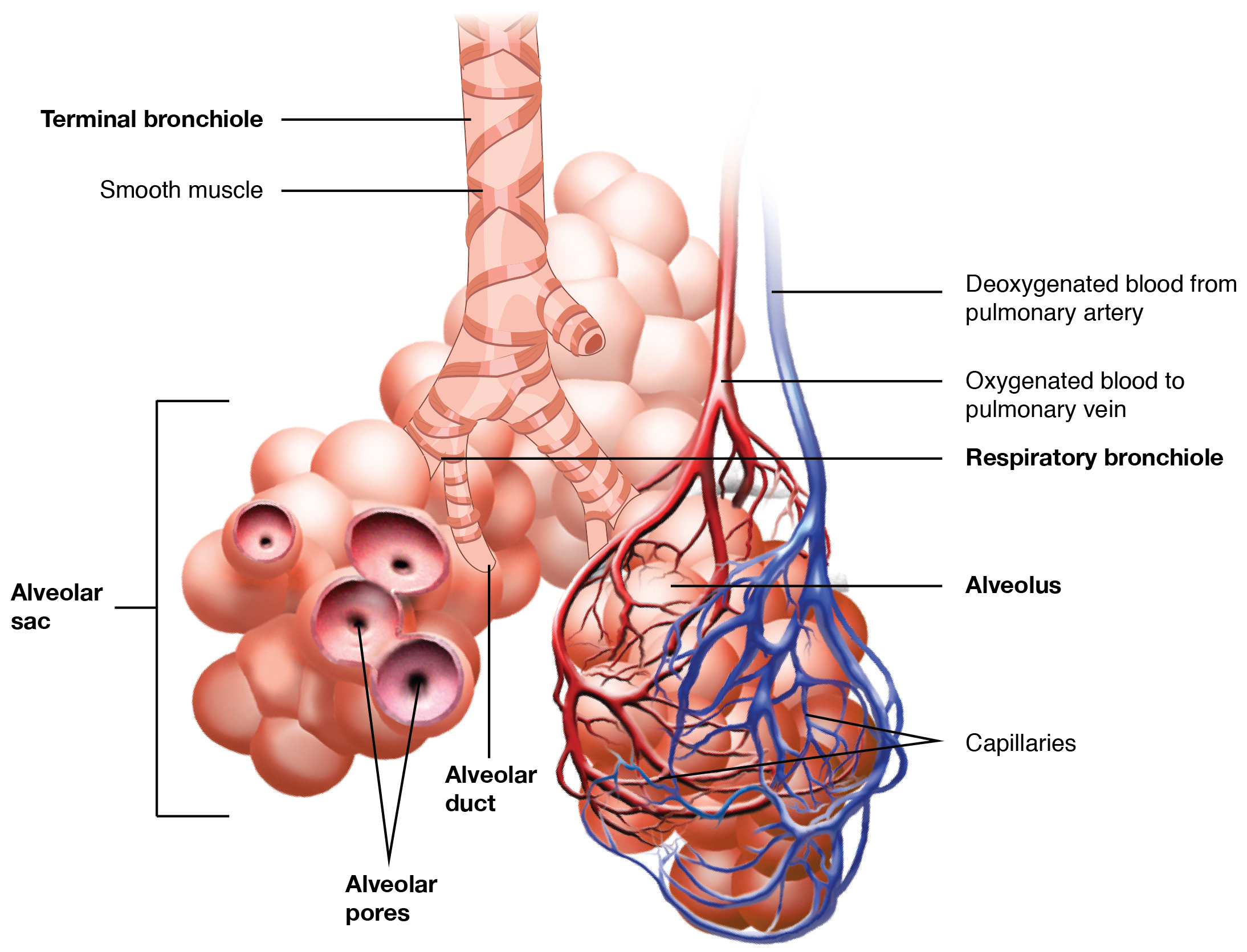
Alveoli
An alveolar duct is a tube composed of smooth muscle and connective tissue, which opens into a cluster of alveoli. An alveolus is one of the many small, grape-like sacs that are attached to the alveolar ducts.
An alveolar sac is a cluster of many individual alveoli that are responsible for gas exchange. An alveolus is approximately 200 μm in diameter with elastic walls that allow the alveolus to stretch during air intake, increasing the surface area available for gas exchange. Alveoli are connected to their neighbors by alveolar pores, which help maintain equal air pressure throughout the alveoli and lung.
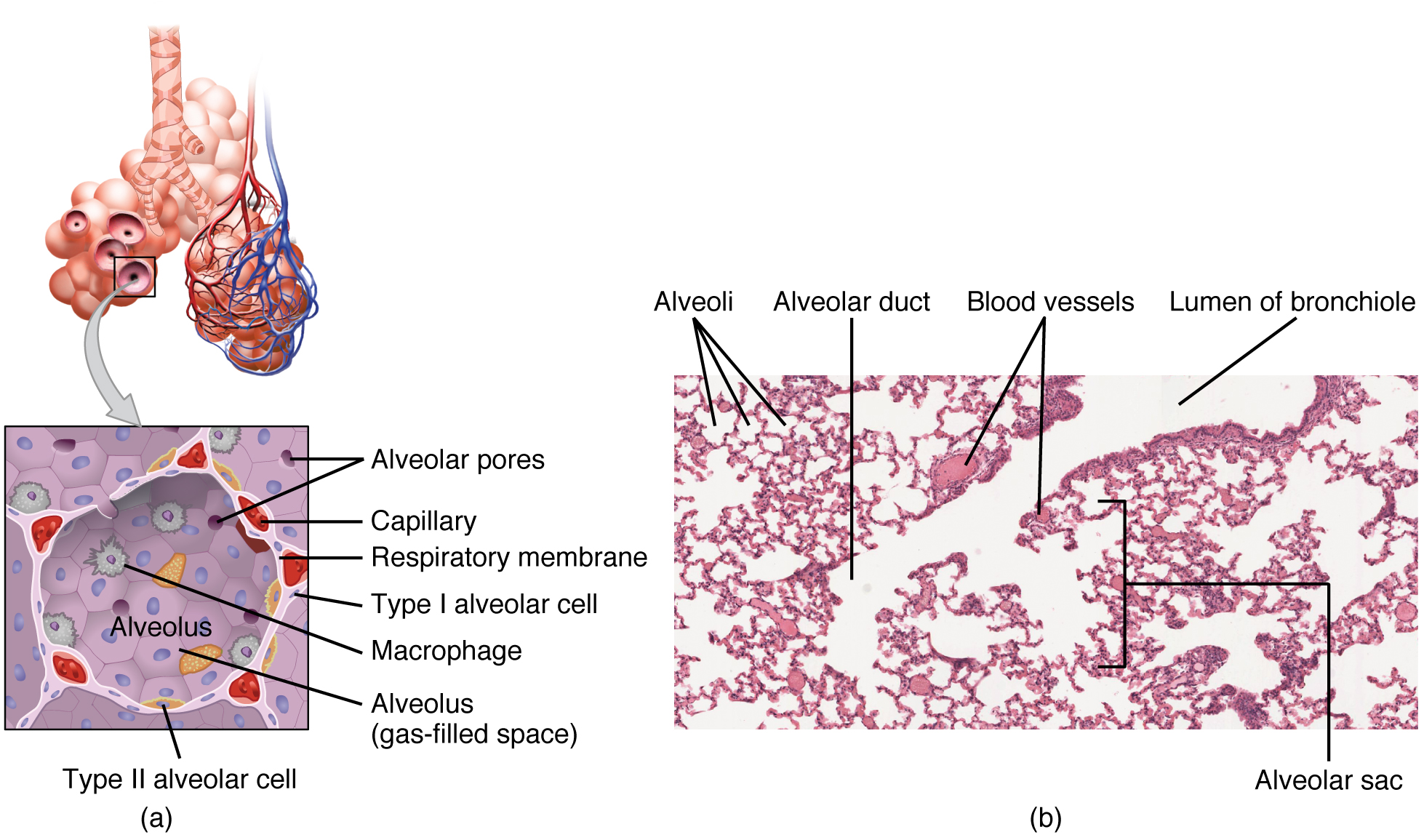
The alveolar wall consists of three major cell types: type I alveolar cells, type II alveolar cells, and alveolar macrophages. A type I alveolar cell is a squamous epithelial cell of the alveoli, which constitutes up to 97 percent of the alveolar surface area. These cells are about 25 nm thick and are highly permeable to gases. A type II alveolar cell is interspersed among the type I cells and secretes pulmonary surfactant, a substance composed of phospholipids and proteins that reduces the surface tension of the alveoli. Roaming around the alveolar wall is the alveolar macrophage, a phagocytic cell of the immune system that removes debris and pathogens that have reached the alveoli.
The simple squamous epithelium formed by type I alveolar cells is attached to a thin, elastic basement membrane. This epithelium is extremely thin and borders the endothelial membrane of capillaries. Taken together, the alveoli and capillary membranes form a respiratory membrane that is approximately 0.5 μm (micrometers) thick. The respiratory membrane allows gases to cross by simple diffusion, allowing oxygen to be picked up by the blood for transport and CO2 to be released into the air of the alveoli.
Asthma – FYI (For Your Information -> you will not be assessed on this content)
Asthma is a common condition that affects the lungs in both adults and children. Approximately 8.2 percent of adults (18.7 million) and 9.4 percent of children (7 million) in the United States have asthma. Also, asthma is the most frequent cause of hospitalization in children.
Asthma is a chronic disease characterized by inflammation and edema of the airway, and bronchospasms (that is, constriction of the bronchioles), which can inhibit air from entering the lungs. Also, excessive mucus secretion can occur, which further contributes to airway occlusion. Cells of the immune system, such as eosinophils and mononuclear cells, may also be involved in infiltrating the walls of the bronchi and bronchioles.
Bronchospasms occur periodically and lead to an “asthma attack.” An attack may be triggered by environmental factors such as dust, pollen, pet hair, or dander, changes in the weather, mold, tobacco smoke, and respiratory infections, or by exercise and stress.
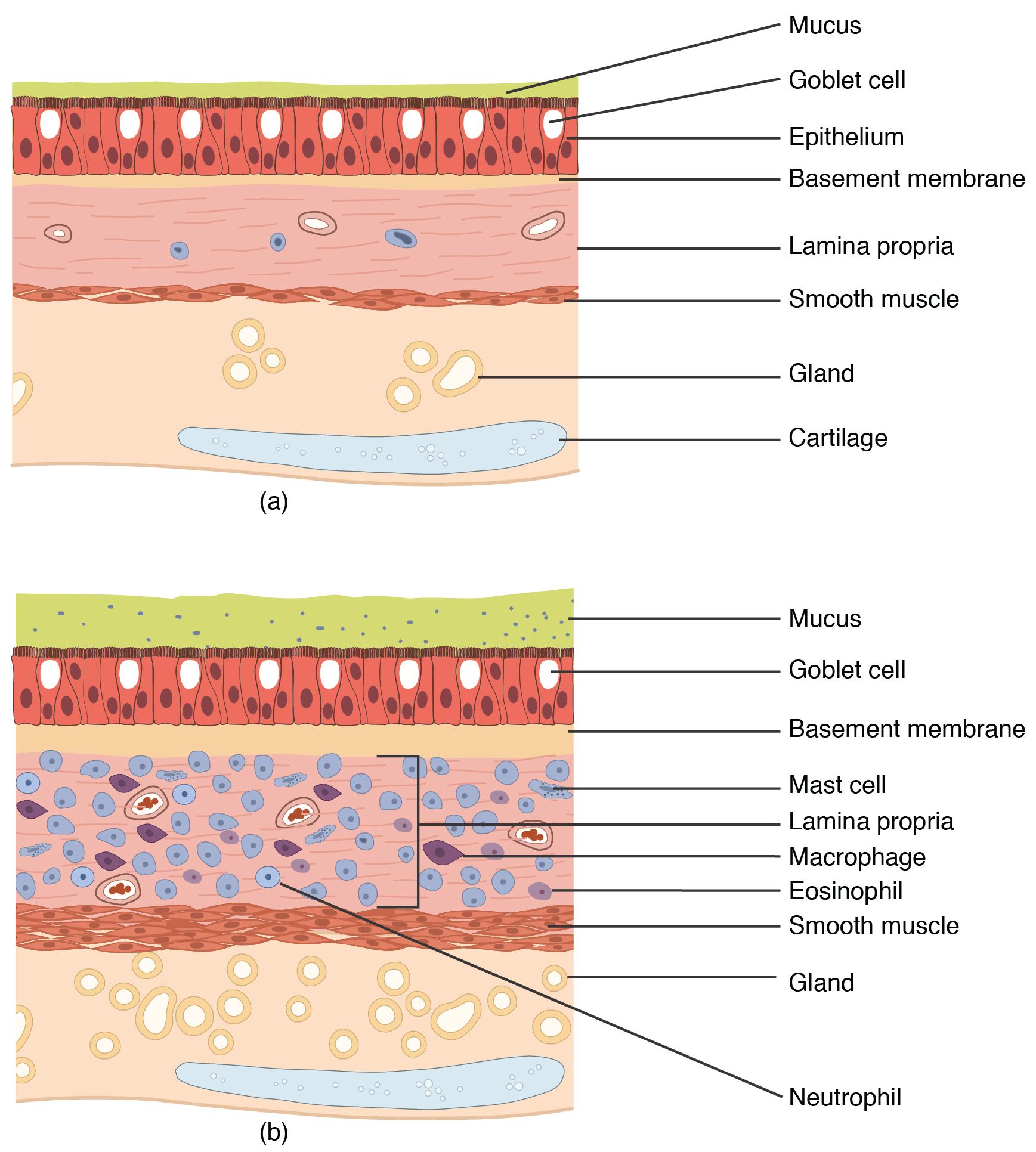
Symptoms of an asthma attack involve coughing, shortness of breath, wheezing, and tightness of the chest. Symptoms of a severe asthma attack that requires immediate medical attention would include difficulty breathing that results in blue (cyanotic) lips or face, confusion, drowsiness, a rapid pulse, sweating, and severe anxiety. The severity of the condition, frequency of attacks, and identified triggers influence the type of medication that an individual may require. Longer-term treatments are used for those with more severe asthma. Short-term, fast-acting drugs that are used to treat an asthma attack are typically administered via an inhaler. For young children or individuals who have difficulty using an inhaler, asthma medications can be administered via a nebulizer.
The Lungs
*This content is covered in the pre-lecture assignment and will not be covered again in lecture: Gross Anatomy of the Lungs.
*This content is introduced in the pre-lecture assignment and will be elaborated on in lecture: pleura.
*This content will be covered in lecture: blood supply, innervation.
A major organ of the respiratory system, each lung houses structures of both the conducting and respiratory zones. The lungs’ main function is to perform the exchange of oxygen and carbon dioxide with air from the atmosphere. To this end, the lungs exchange respiratory gases across an extensive epithelial surface area—about 70 square meters—that is highly permeable to gases.
Gross Anatomy of the Lungs
The lungs are pyramid-shaped, paired organs connected to the trachea by the right and left bronchi; on the inferior surface, the lungs are bordered by the diaphragm. The diaphragm is the flat, dome-shaped muscle located at the base of the lungs and thoracic cavity. The lungs are enclosed by the pleurae, which are attached to the mediastinum. The right lung is shorter and wider than the left lung, and the left lung occupies a smaller volume than the right. The cardiac notch is an indentation on the left lung’s surface, and it allows space for the heart. The apex of the lung is the superior region, whereas the base is the opposite region near the diaphragm. The costal surface of the lung borders the ribs. The mediastinal surface faces the midline.

Each lung is composed of smaller units called lobes. Fissures separate these lobes from each other. The right lung consists of three lobes: the superior, middle, and inferior lobes, and therefore as two fissures. The oblique fissure, separating the inferior and middle lobes, and the horizontal fissure, separating the middle and superior lobes. The left lung consists of two lobes: the superior and inferior lobes, and has one fissure called the oblique fissure. A bronchopulmonary segment is a division of a lobe, and each lobe houses multiple bronchopulmonary segments. Each segment receives air from its own tertiary bronchus and is supplied with blood by its own artery. Some diseases of the lungs typically affect one or more bronchopulmonary segments, and in some cases, the diseased segments can be surgically removed with little influence on neighboring segments. A pulmonary lobule is a subdivision formed as the bronchi branch into bronchioles. Each lobule receives its own large bronchiole that has multiple branches. An interlobular septum is a wall composed of connective tissue, which separates lobules from one another.
Blood Supply and Nervous Innervation of the Lungs
The blood supply of the lungs plays an important role in gas exchange and serves as a transport system for gases throughout the body. Also, innervation by both the parasympathetic and sympathetic nervous systems provides an important level of control through dilation and constriction of the airway.
Blood Supply
The major function of the lungs is to perform gas exchange, which requires blood from the pulmonary circulation. This blood supply contains deoxygenated blood and travels to the lungs, where erythrocytes, also known as red blood cells, pick up oxygen to be transported to tissues throughout the body. The pulmonary artery is an artery that arises from the pulmonary trunk and carries deoxygenated, arterial blood to the alveoli. The pulmonary artery branches multiple times as it follows the bronchi, and each branch becomes progressively smaller in diameter. One arteriole and an accompanying venule supply and drain one pulmonary lobule. As they near the alveoli, the pulmonary arteries become the pulmonary capillary network. The pulmonary capillary network consists of tiny vessels with very thin walls that lack smooth muscle fibers. The capillaries branch and follow the bronchioles and structure of the alveoli. It is at this point that the capillary wall meets the alveolar wall, creating the respiratory membrane. Once the blood is oxygenated, it drains from the alveoli by way of multiple pulmonary veins, which exit the lungs through the hilum.
Nervous Innervation
Dilation and constriction of the airway are achieved through nervous control by the parasympathetic and sympathetic nervous systems. The parasympathetic system causes bronchoconstriction, whereas the sympathetic nervous system stimulates bronchodilation. Reflexes such as coughing, and the ability of the lungs to regulate oxygen and carbon dioxide levels, also result from this autonomic nervous system control. Sensory nerve fibers arise from the vagus nerve and from the second to fifth thoracic ganglia. The pulmonary plexus is a region on the lung root formed by the entrance of the nerves at the hilum. The nerves then follow the bronchi in the lungs and branch to innervate muscle fibers, glands, and blood vessels.
Pleura of the Lungs
Each lung is enclosed within a cavity that is surrounded by the pleura. The pleura (plural = pleurae) is a serous membrane that surrounds the lung. The right and left pleurae, which enclose the right and left lungs, are separated by the mediastinum. The pleurae consist of two layers. The visceral pleura is the layer that is superficial to the lungs and extends into and lines the lung fissures. In contrast, the parietal pleura is the outer layer that connects to the thoracic wall, the mediastinum, and the diaphragm. The visceral and parietal pleurae connect at the hilum. The pleural cavity is the space between the visceral and parietal layers.
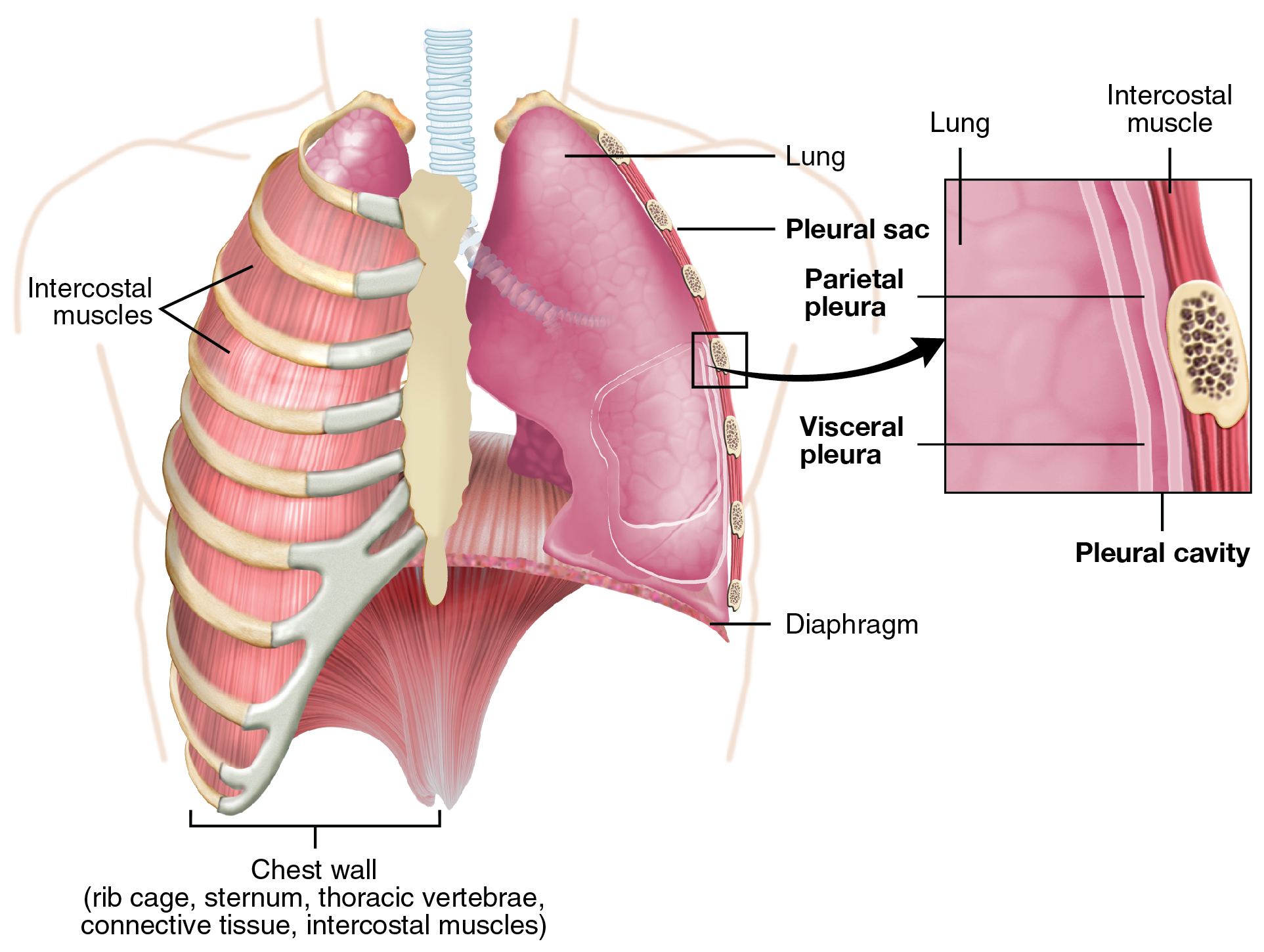
The pleurae perform two major functions: They produce pleural fluid and create cavities that separate the major organs. Pleural fluid is secreted by mesothelial cells from both pleural layers and acts to lubricate their surfaces. This lubrication reduces friction between the two layers to prevent trauma during breathing and creates surface tension that helps maintain the position of the lungs against the thoracic wall. This adhesive characteristic of the pleural fluid causes the lungs to enlarge when the thoracic wall expands during ventilation, allowing the lungs to fill with air. The pleurae also create a division between major organs that prevents interference due to the movement of the organs while preventing the spread of infection.
The parietal layers of the membranes line the walls of the body cavity (pariet- refers to a cavity wall). The visceral layer of the membrane covers the organs (the viscera). Between the parietal and visceral layers is a very thin, fluid-filled serous space, or cavity. The serous membranes form fluid-filled sacs, or cavities, meant to cushion and reduce friction on internal organs when they move, such as when the lungs inflate or the heart beats. Both the parietal and visceral serosa secrete the thin, slippery serous fluid located within the serous cavities.
The pleura is the serous membrane that encloses the pleural cavity; the pleural cavity surrounds the lungs. The pleural cavity reduces friction between the lungs and the body wall.
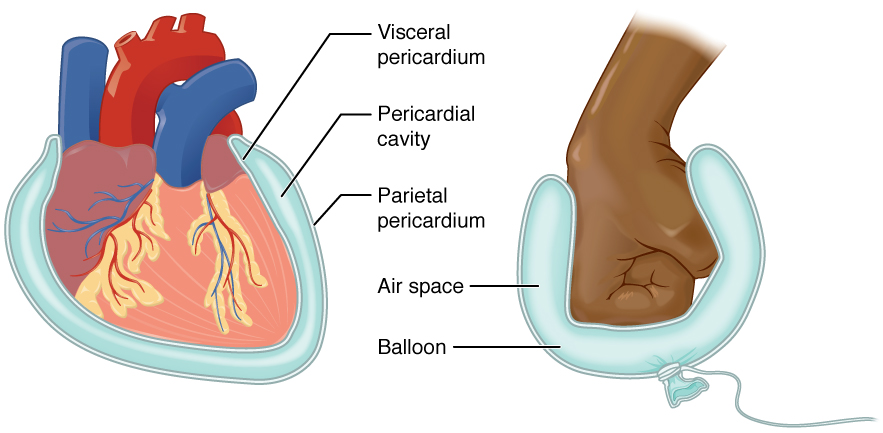
Respiration
*This content is introduced in the pre-lecture assignment and will be elaborated on in lecture.
Pulmonary ventilation is the act of breathing, which can be described as air movement into and out of the lungs. The major mechanisms that drive pulmonary ventilation are atmospheric pressure (Patm); the air pressure within the alveoli, called intra-alveolar pressure (Palv); and the pressure within the pleural cavity, called intrapleural pressure (Pip).
Mechanisms of Breathing
The intra-alveolar and intrapleural pressures are dependent on certain physical features of the lung. However, the ability to breathe—to have air enter the lungs during inspiration and air leaves the lungs during expiration—is dependent on the air pressure of the atmosphere and the air pressure within the lungs.
Pressure Relationships
Inspiration (or inhalation) and expiration (or exhalation) are dependent on the differences in pressure between the atmosphere and the lungs. In a gas, pressure is a force created by the movement of gas molecules that are confined. Boyle’s Law describes the relationship between volume and pressure in a gas at a constant temperature. Boyle discovered that the pressure of a gas is inversely proportional to its volume: if volume increases, pressure decreases. Likewise, if volume decreases, pressure increases. Pressure and volume are inversely related (P = k/V).
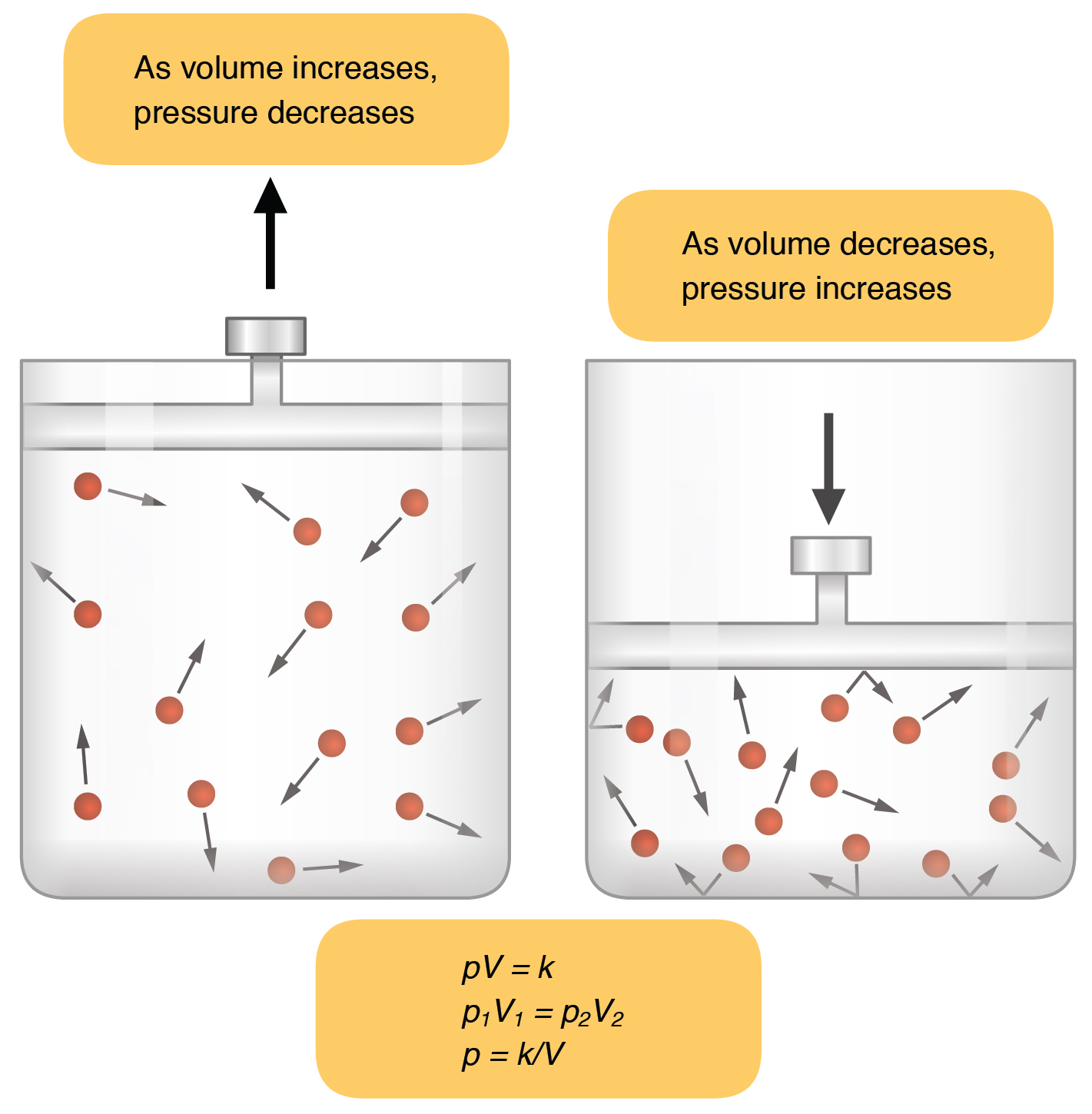
Pressure Relationships – FYI (For Your Information -> you will not be assessed on this content)
Pulmonary ventilation is dependent on three types of pressure: atmospheric, intra-alveolar, and intrapleural. Atmospheric pressure is the amount of force exerted by gases in the air surrounding any given surface, such as the body. Atmospheric pressure can be expressed in terms of the unit atmosphere, abbreviated atm, or in millimeters of mercury (mm Hg). One atm is equal to 760 mm Hg, which is the atmospheric pressure at sea level. Typically, for respiration, other pressure values are discussed in relation to atmospheric pressure. Therefore, negative pressure is a pressure lower than the atmospheric pressure, whereas positive pressure is the pressure that is greater than the atmospheric pressure. A pressure that is equal to the atmospheric pressure is expressed as zero.
Intra-alveolar pressure (intrapulmonary pressure) is the pressure of the air within the alveoli, which changes during the different phases of breathing ((Figure)). Because the alveoli are connected to the atmosphere via the tubing of the airways (similar to the two- and one-liter containers in the example above), the intrapulmonary pressure of the alveoli always equalizes with the atmospheric pressure.
Intra-alveolar pressure changes during the different phases of the cycle. It equalizes at 760 mm Hg but does not remain at 760 mm Hg.
Intrapleural pressure is the pressure of the air within the pleural cavity, between the visceral and parietal pleurae. Similar to intra-alveolar pressure, intrapleural pressure also changes during the different phases of breathing. However, due to certain characteristics of the lungs, the intrapleural pressure is always lower than, or negative to, the intra-alveolar pressure (and atmospheric pressure). Although it fluctuates during inspiration and expiration, intrapleural pressure remains approximately –4 mm Hg throughout the breathing cycle.
Competing forces within the thorax cause the formation of the negative intrapleural pressure. One of these forces relates to the elasticity of the lungs themselves—elastic tissue pulls the lungs inward, away from the thoracic wall. Surface tension of alveolar fluid, which is mostly water, also creates an inward pull of the lung tissue. This inward tension from the lungs is countered by opposing forces from the pleural fluid and thoracic wall. Surface tension within the pleural cavity pulls the lungs outward. Too much or too little pleural fluid would hinder the creation of the negative intrapleural pressure; therefore, the level must be closely monitored by the mesothelial cells and drained by the lymphatic system. Since the parietal pleura is attached to the thoracic wall, the natural elasticity of the chest wall opposes the inward pull of the lungs. Ultimately, the outward pull is slightly greater than the inward pull, creating the –4 mm Hg intrapleural pressure relative to the intra-alveolar pressure. Transpulmonary pressure is the difference between the intrapleural and intra-alveolar pressures, and it determines the size of the lungs. A higher transpulmonary pressure corresponds to a larger lung.
Physical Factors Affecting Ventilation
In addition to the differences in pressures, breathing depends on the contraction and relaxation of muscle fibers of both the diaphragm and thorax. The lungs themselves are passive during breathing, meaning they are not involved in creating the movement that helps inspiration and expiration. This is because of the adhesive nature of the pleural fluid, which allows the lungs to be pulled outward when the thoracic wall moves during inspiration. The recoil of the thoracic wall during expiration causes compression of the lungs. Contraction and relaxation of the diaphragm and intercostals muscles (found between the ribs) cause most of the pressure changes that result in inspiration and expiration. These muscle movements and subsequent pressure changes cause air to either rush in or be forced out of the lungs.
As noted earlier, there is surface tension within the alveoli caused by water present in the lining of the alveoli. This surface tension tends to inhibit the expansion of the alveoli. However, pulmonary surfactant secreted by type II alveolar cells mixes with that water and helps reduce this surface tension. Without pulmonary surfactant, the alveoli would collapse during expiration.
Thoracic wall compliance is the ability of the thoracic wall to stretch while under pressure. This can also affect the effort expended in the process of breathing. For inspiration to occur, the thoracic cavity must expand. The expansion of the thoracic cavity directly influences the capacity of the lungs to expand. If the thoracic wall tissues are not very compliant, it will be difficult to expand the thorax to increase the size of the lungs.
Pulmonary Ventilation
The difference in pressures drives pulmonary ventilation because air flows down a pressure gradient. That is, air flows from an area of higher pressure to an area of lower pressure. Air flows into the lungs largely due to a pressure difference; atmospheric pressure is greater than intra-alveolar pressure, and intra-alveolar pressure is greater than intrapleural pressure. Air flows out of the lungs during expiration based on the same principle; pressure within the lungs becomes greater than the atmospheric pressure.
Pulmonary ventilation comprises two major steps: inspiration and expiration. Inspiration is the process that causes air to enter the lungs, and expiration is the process that causes air to leave the lungs. In general, two muscle groups are used during normal inspiration: the diaphragm and the external intercostal muscles. Additional muscles can be used if a bigger breath is required. When the diaphragm contracts, it moves inferiorly toward the abdominal cavity, creating a larger thoracic cavity and more space for the lungs. Contraction of the external intercostal muscles moves the ribs upward and outward, causing the rib cage to expand, which increases the volume of the thoracic cavity. Due to the pleural fluid’s adhesive force, the expansion of the thoracic cavity forces the lungs to stretch and expand as well. This increase in volume leads to decreased intra-alveolar pressure, creating a pressure lower than atmospheric pressure. As a result, a pressure gradient is created that drives air into the lungs.
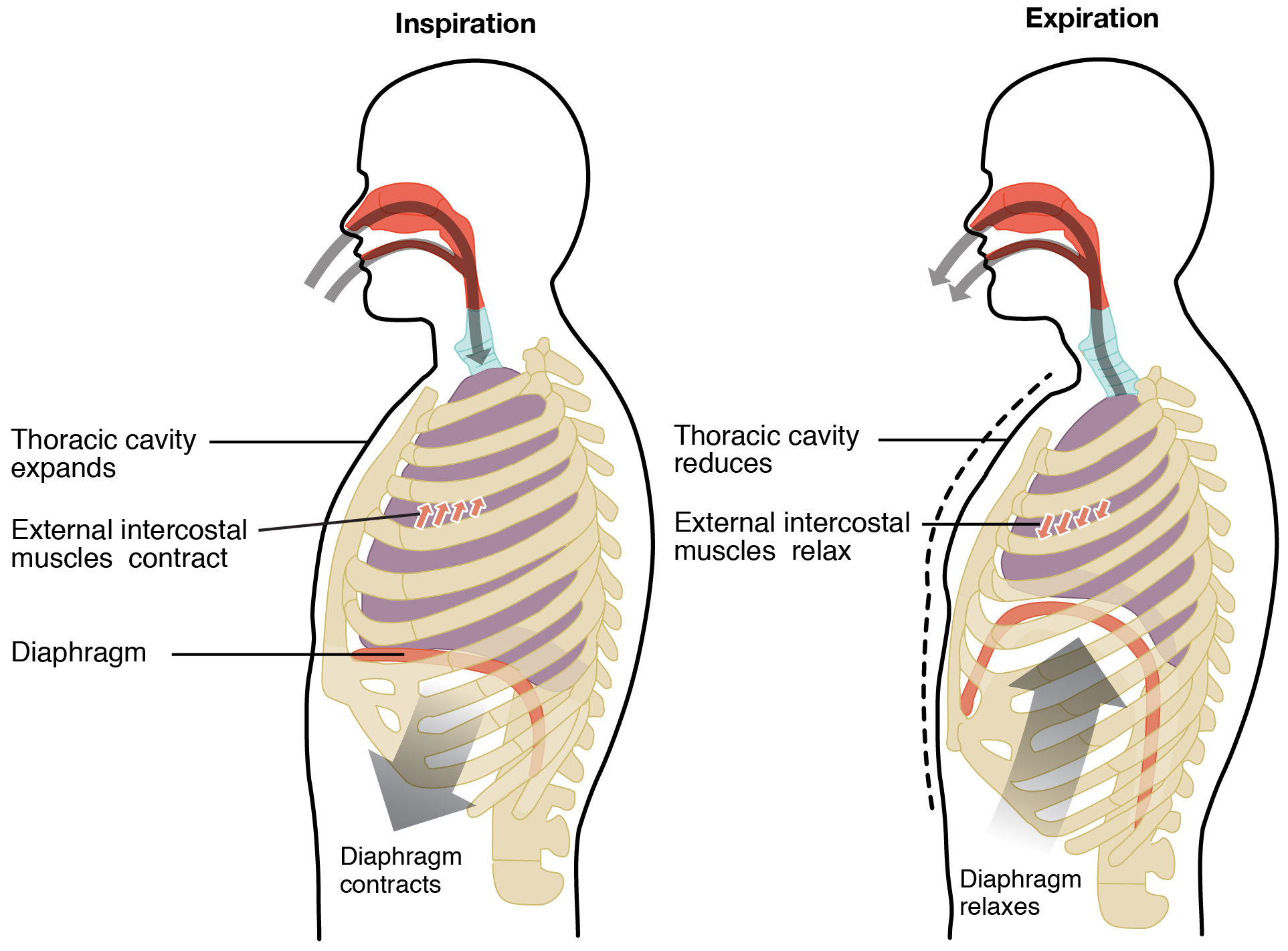
The process of normal expiration is passive, meaning that energy is not required to push air out of the lungs. Instead, the lung tissue’s elasticity causes the lung to recoil as the diaphragm and intercostal muscles relax following inspiration. In turn, the thoracic cavity and lungs decrease in volume, causing an increase in intrapulmonary pressure. The intrapulmonary pressure rises above atmospheric pressure, creating a pressure gradient that causes air to leave the lungs.
There are different types, or modes, of breathing that require a slightly different process to allow inspiration and expiration. Quiet breathing is a mode of breathing that occurs at rest and does not require the cognitive thought of the individual. During passive inspiration, the diaphragm and external intercostals must contract. Passive expiration does not require muscle to contract.
A deep breath, called diaphragmatic breathing, requires the diaphragm to contract. As the diaphragm relaxes, air passively leaves the lungs. A shallow breath, called costal breathing, requires contraction of the intercostal muscles. As the intercostal muscles relax, air passively leaves the lungs.
In contrast, forced breathing is a mode of breathing that can occur during exercise or actions that require the active manipulation of breathing, such as singing. During forced breathing, inspiration and expiration both occur due to muscle contractions. In addition to the contraction of the diaphragm and intercostal muscles, other accessory muscles must also contract. During forced inspiration, muscles of the neck, including the scalenes, contract and lift the thoracic wall, increasing lung volume. During forced expiration, accessory muscles of the abdomen contract, forcing abdominal organs upward against the diaphragm. This helps to push the diaphragm further into the thorax, pushing more air out. Also, accessory muscles (primarily the internal intercostals) help compress the rib cage, reducing the volume of the thoracic cavity.
Muscles of the Thorax – Respiration
*This content is introduced in the pre-lecture assignment and will be elaborated on in lecture.
The muscles of the chest serve to facilitate breathing by changing the size of the thoracic cavity. When you inhale, your chest rises because the cavity expands. Alternately, when you exhale, your chest falls because the thoracic cavity decreases in size.
We will discuss the mechanics of respiration further in the videos posted in Canvas. The serratus posterior superior, serratus posterior inferior, and transverus thoracis are presented in the lecture slides and videos posted in Canvas.
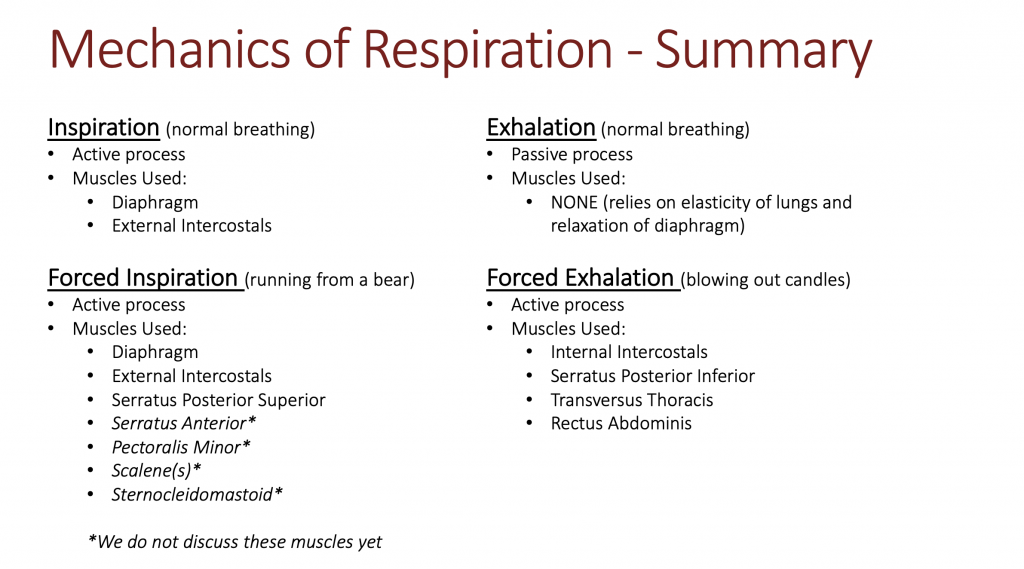
The Diaphragm
The change in volume of the thoracic cavity during breathing is due to the diaphragm’s alternate contraction and relaxation. It separates the thoracic and abdominal cavities and is dome-shaped at rest. The superior surface of the diaphragm is convex, creating the elevated floor of the thoracic cavity. The inferior surface is concave, creating the curved roof of the abdominal cavity.
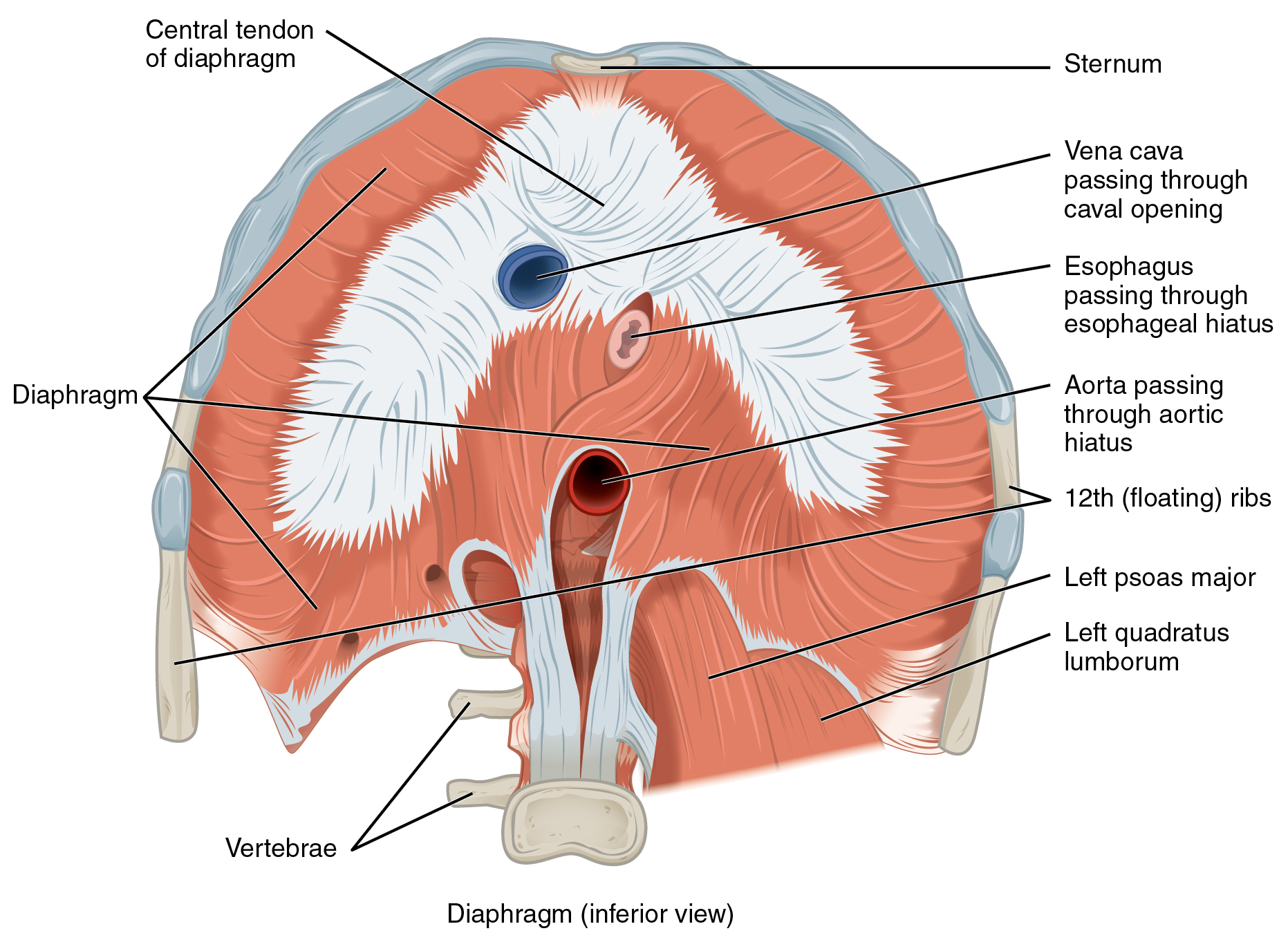
Defecating, urination, and even childbirth involve cooperation between the diaphragm and abdominal muscles (this cooperation is referred to as the “Valsalva maneuver”). You hold your breath by a steady contraction of the diaphragm; this stabilizes the peritoneal cavity’s volume and pressure. When the abdominal muscles contract, the pressure cannot push the diaphragm up, so it increases pressure on the intestinal tract (defecation), urinary tract (urination), or reproductive tract (childbirth).
The inferior surface of the pericardial sac and the inferior surfaces of the pleural membranes (parietal pleura) fuse onto the central tendon of the diaphragm. To the sides of the tendon are the skeletal muscle portions of the diaphragm, which insert into the tendon while having several origins, including the xiphoid process of the sternum anteriorly, the inferior six ribs and their cartilages laterally, and the lumbar vertebrae and 12th ribs posteriorly.
The diaphragm also includes three openings for the passage of structures between the thorax and the abdomen. The inferior vena cava passes through the caval opening, and the esophagus and attached nerves pass through the esophageal hiatus. The aorta, thoracic duct, and azygous vein pass through the aortic hiatus of the posterior diaphragm.
The Intercostal Muscles
Some muscles span each of the intercostal spaces. The principal role of the intercostal muscles is to assist in breathing by changing the dimensions of the rib cage.
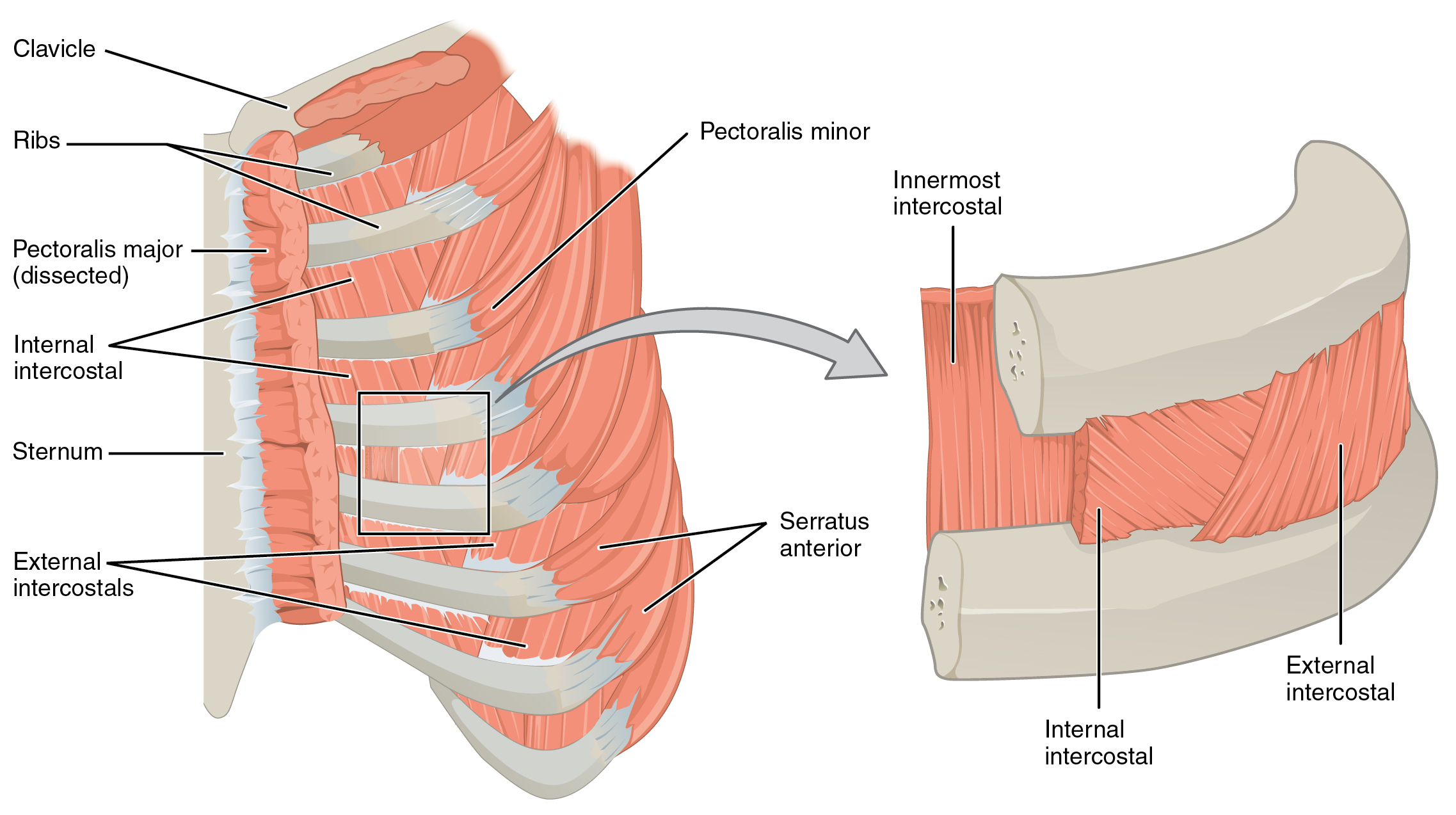
The 11 pairs of superficial external intercostal muscles aid in the inspiration of air during breathing because when they contract, they raise the rib cage, which expands it. The 11 pairs of internal intercostal muscles, just under the externals, are used for expiration because they draw the ribs together to constrict the rib cage.
Fun Fact – Respiratory Adjustments (-> you will not be assessed on this content)
Although the fetus “practices” breathing by inhaling amniotic fluid in utero, there is no air in the uterus and thus no true opportunity to breathe. (There is also no need to breathe because the placenta supplies the fetus with all the oxygenated blood it needs.) During gestation, the partially collapsed lungs are filled with amniotic fluid and exhibit very little metabolic activity. Several factors stimulate newborns to take their first breath at birth. First, labor contractions temporarily constrict umbilical blood vessels, reducing oxygenated blood flow to the fetus and elevating carbon dioxide levels in the blood. High carbon dioxide levels cause acidosis and stimulate the respiratory center in the brain, triggering the newborn to take a breath.
The first breath typically is taken within 10 seconds of birth, after mucus is aspirated from the infant’s mouth and nose. The first breaths inflate the lungs to nearly full capacity and dramatically decrease lung pressure and resistance to blood flow, causing a major circulatory reconfiguration. Pulmonary alveoli open, and alveolar capillaries fill with blood. Amniotic fluid in the lungs drains or is absorbed, and the lungs immediately take over the task of the placenta, exchanging carbon dioxide for oxygen by the process of respiration.