Module 16: Urinary System
Learning Objectives:
By the end of this module, students will be able to:
- Explain the functions of the urinary system.
- Describe the gross and microscopic anatomy of the kidneys.
- List the components of the nephron, and explain their roles in urine formation.
- Describe the anatomy, location, and function of the ureters, urinary bladder, and male and female urethras.
- List the blood vessels supplying the structures of the urinary system.
Terms to Know
Kidney
|
Kidney (continued)
Urinary Tract
General
|
The Kidneys
The kidneys lie on either side of the spine in the retroperitoneal space between the parietal peritoneum and the posterior abdominal wall, well protected by muscle, fat, and ribs. They are roughly the size of your fist, and the male kidney is typically a bit larger than the female kidney. The kidneys are well vascularized, receiving about 25 percent of the cardiac output at rest.
External Anatomy
The left kidney is located at about the T12 to L3 vertebrae, whereas the right is lower due to slight displacement by the liver. The eleventh and twelfth ribs somewhat protect the upper portions of the kidneys. They are about 11–14 cm in length, 6 cm wide, and 4 cm thick, and are directly covered by a fibrous capsule composed of dense, irregular connective tissue that helps to hold their shape and protect them. This capsule is covered by a shock-absorbing layer of adipose tissue called the renal fat pad, which is encompassed by a tough renal fascia. To a lesser extent, the fascia and the overlying peritoneum serve to firmly anchor the kidneys to the posterior abdominal wall in a retroperitoneal position. On the superior aspect of each kidney is the adrenal gland.
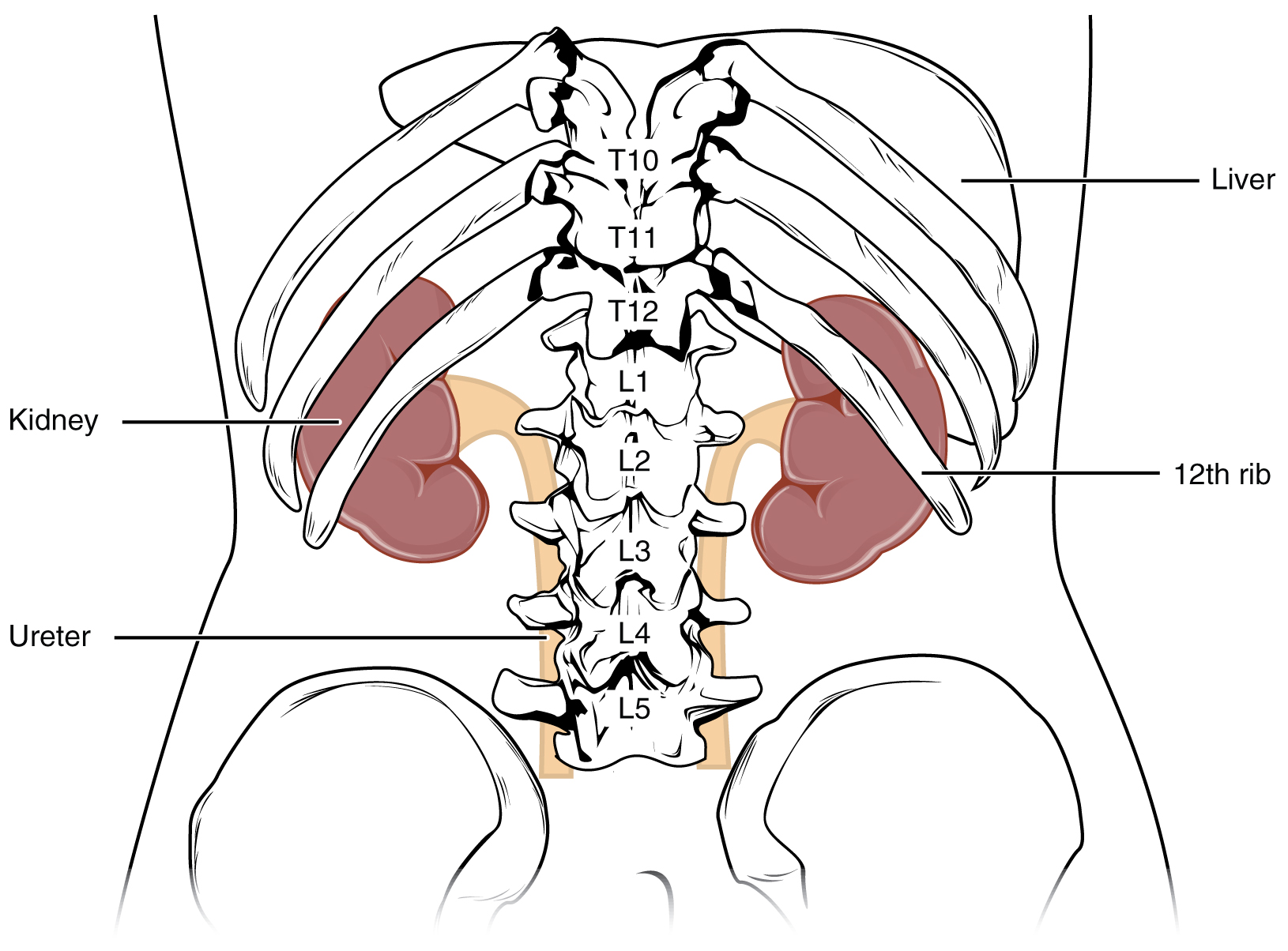
Internal Anatomy
A frontal section through the kidney reveals an outer region called the renal cortex and an inner region called the medulla. The renal columns are connective tissue extensions that radiate downward from the cortex through the medulla to separate the medulla’s most characteristic features, the renal pyramids. The papillae are bundles of collecting ducts that transport urine made by nephrons to the calyces of the kidney for excretion. The renal columns also serve to divide the kidney into 6–8 lobes and provide a supportive framework for vessels that enter and exit the cortex.
Renal Hilum
The renal hilum is the entry and exit site for structures servicing the kidneys: vessels, nerves, lymphatics, and ureters. The medial-facing hila are tucked into the sweeping convex outline of the cortex. Emerging from the hilum is the renal pelvis, formed from the major and minor calyxes in the kidney. The smooth muscle in the renal pelvis funnels urine via peristalsis into the ureter. The renal arteries form directly from the descending aorta, whereas the renal veins return cleansed blood directly to the inferior vena cava. The artery, vein, and renal pelvis are arranged in an anterior-to-posterior order.
Nephrons and Vessels
The renal artery first divides into segmental arteries, followed by further branching to form interlobar arteries that pass through the renal columns to reach the cortex. In turn, the interlobar arteries branch into arcuate arteries, cortical radiate arteries, and then into afferent arterioles. The afferent arterioles service about 1.3 million nephrons in each kidney.
Nephrons are the “functional units” of the kidney; they cleanse the blood and balance the constituents of the circulation. The afferent arterioles form a tuft of high-pressure capillaries about 200 µm in diameter, the glomerulus. The rest of the nephron consists of a continuous sophisticated tubule whose proximal end surrounds the glomerulus in an intimate embrace—this is the glomerular (Bowman’s) capsule. The glomerulus and glomerular capsule together form the renal corpuscle. As mentioned earlier, these glomerular capillaries filter the blood based on particle size. After passing through the renal corpuscle, the capillaries form a second arteriole, the efferent arteriole. Next, these will form a capillary network around the more distal portions of the nephron tubule, the peritubular capillaries, and the vasa recta before returning to the venous system. As the glomerular filtrate progresses through the nephron, these capillary networks recover most of the solutes and water and return them to circulation. Since a capillary bed (the glomerulus) drains into a vessel that in turn forms a second capillary bed, the definition of a portal system is met. This is the only portal system in which an arteriole is found between the first and second capillary beds. (Portal systems also link the hypothalamus to the anterior pituitary and the blood vessels of the digestive viscera to the liver).
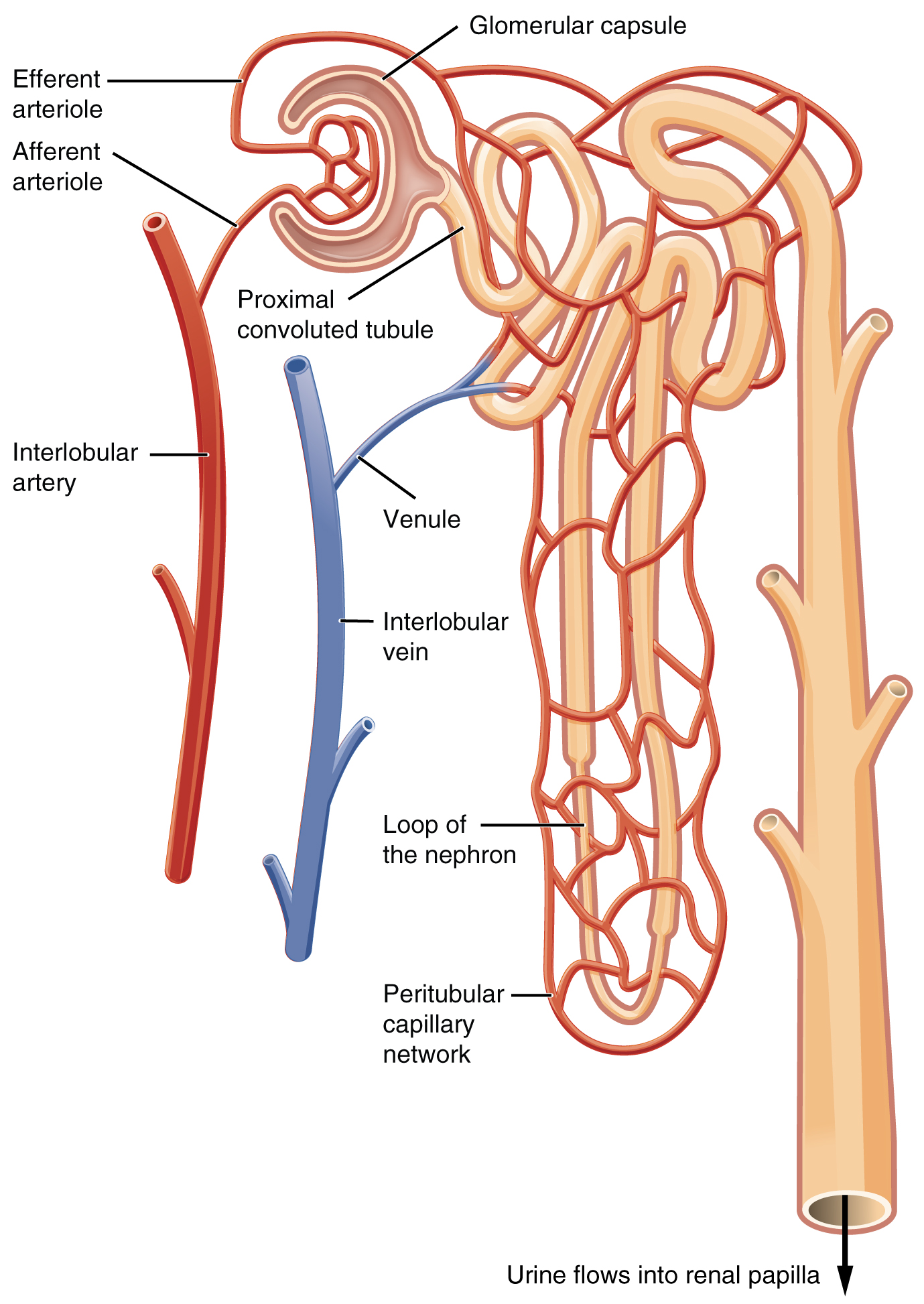
Cortex
It is easy to identify the cortex; it appears lighter in color compared to the rest of the kidney. All of the renal corpuscles and both the proximal convoluted tubules (PCTs) and distal convoluted tubules are found here. Some nephrons have a short loop of Henle that does not dip beyond the cortex. These nephrons are called cortical nephrons. About 15 percent of nephrons have long loops of Henle that extend deep into the medulla and are called juxtamedullary nephrons.
The naked eye cannot see the renal structures that conduct the kidney’s essential work. Only a light or electron microscope can reveal these structures. Even then, serial sections and computer reconstruction are necessary to give us a comprehensive view of the nephron’s functional anatomy and its associated blood vessels.
Nephrons: The Functional Unit
Nephrons take a simple filtrate of the blood and modify it into the urine. Many changes take place in the different parts of the nephron before the urine is created for disposal. The term forming urine will be used hereafter to describe the filtrate as it is modified into true urine. The nephron’s primary role is to balance the plasma to homeostatic set points and excrete potential toxins in the urine. They do this by accomplishing three principle functions—filtration, reabsorption, and secretion. They also have additional secondary functions that exert control in three areas: blood pressure (via the production of renin), red blood cell production (via the hormone EPO), and calcium absorption (via conversion of calcidiol into calcitriol, the active form of vitamin D).
Renal Corpuscle
The renal corpuscle consists of a tuft of capillaries called the glomerulus that is largely surrounded by the glomerular capsule. The glomerulus is a high-pressure capillary bed between afferent and efferent arterioles. The glomerular capsule surrounds the glomerulus to form a lumen and captures and directs this filtrate to the PCT. The outermost part of the glomerular capsule, the parietal layer, is a simple squamous epithelium. It transitions onto the glomerular capillaries in an intimate embrace to form the visceral layer of the capsule. Here, the cells are not squamous but uniquely shaped cells (podocytes) extending finger-like arms (pedicels) to cover the glomerular capillaries. These projections interdigitate to form filtration slits, leaving small gaps between the digits to form a sieve. As blood passes through the glomerulus, 10 to 20 percent of the plasma filters between these sieve-like fingers to be captured by the glomerular capsule and funneled to the PCT. Where the fenestrae (windows) in the glomerular capillaries match the spaces between the podocyte “fingers,” the only thing separating the capillary lumen and the lumen of the glomerular capsule is their shared basement membrane. These three features comprise what is known as the filtration membrane. This membrane permits very rapid movement of filtrate from capillary to capsule through pores that are only 70 nm in diameter.
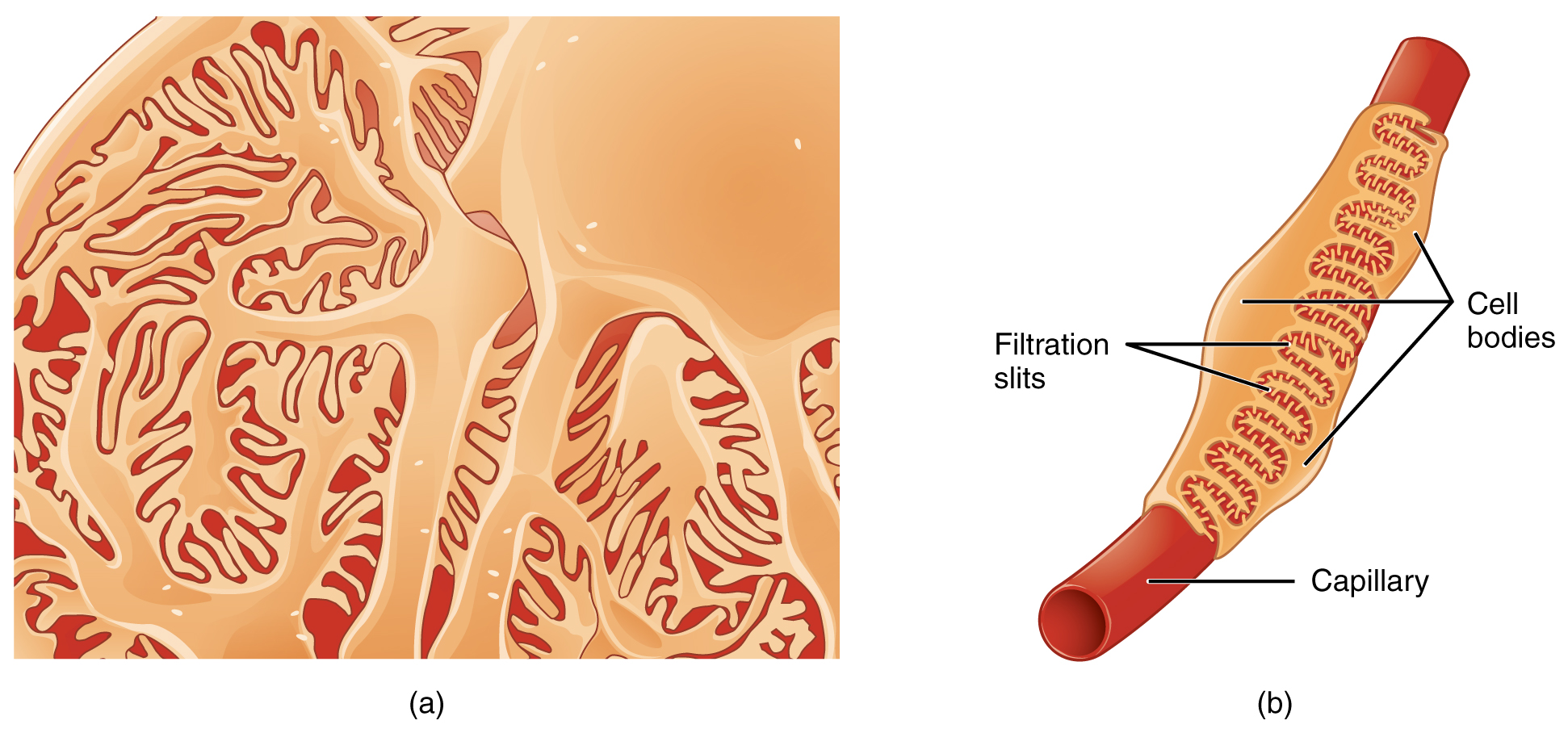
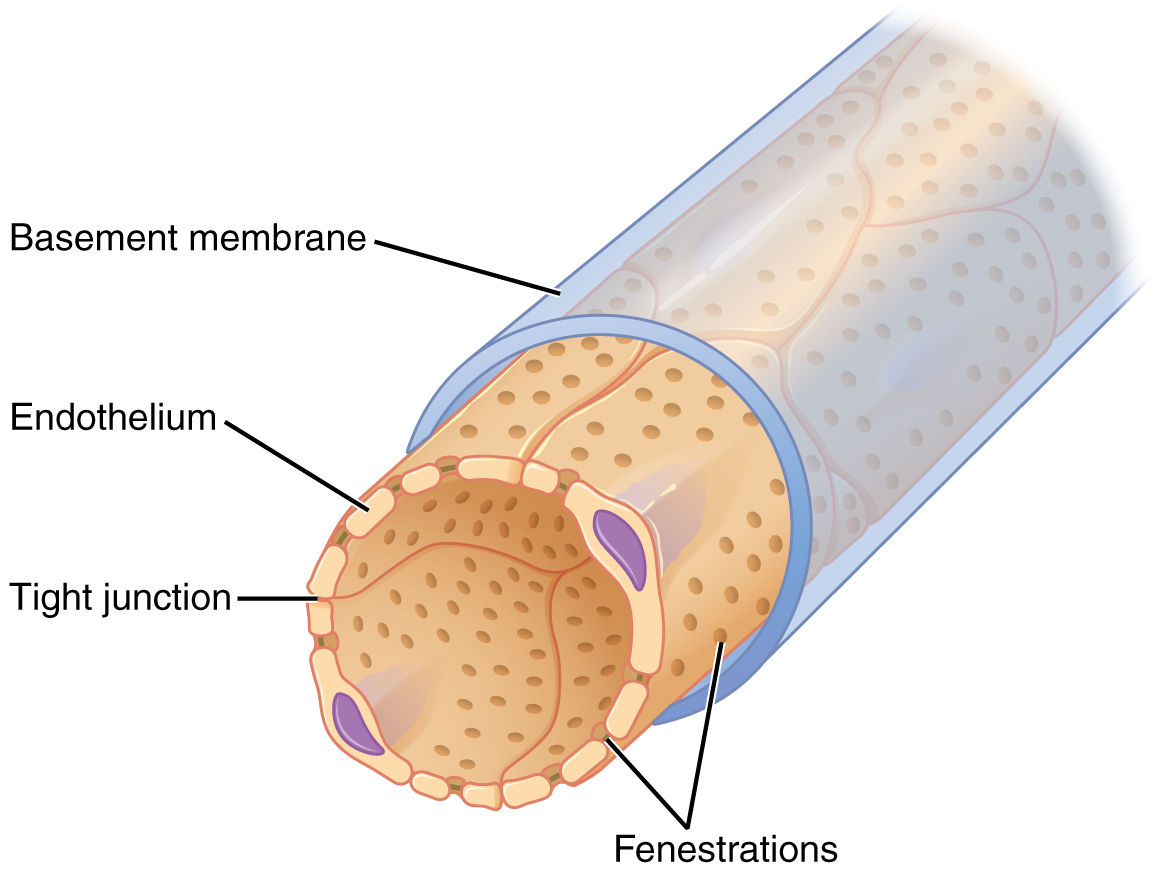
The fenestrations prevent filtration of blood cells or large proteins but allow most other constituents through. These substances cross readily if they are less than 4 nm in size, and most pass freely up to 8 nm. An additional factor affecting the ability of substances to cross this barrier is their electric charge. The proteins associated with these pores are negatively charged, so they tend to repel negatively charged substances and allow positively charged substances to pass more readily. The basement membrane prevents filtration of medium-to-large proteins such as globulins. There are also mesangial cells in the filtration membrane that can contract to help regulate the rate of filtration of the glomerulus. Overall, filtration is regulated by fenestrations in capillary endothelial cells, podocytes with filtration slits, membrane charge, and the basement membrane between capillary cells. The result is the creation of a filtrate that does not contain cells or large proteins and has a slight predominance of positively charged substances.
Lying just outside the glomerular capsule and the glomerulus is the juxtaglomerular apparatus (JGA). At the juncture where the afferent and efferent arterioles enter and leave the glomerular capsule, the initial part of the distal convoluted tubule (DCT) comes into direct contact with the arterioles. At that point, the wall of the DCT forms a part of the JGA known as the macula densa. This cluster of cuboidal epithelial cells monitors the fluid composition of fluid flowing through the DCT. In response to the concentration of Na+ in the fluid flowing past them, these cells release paracrine signals. A second cell type in this apparatus is the juxtaglomerular cell. This is a modified, smooth muscle cell lining the afferent arteriole that can contract or relax in response to ATP or adenosine released by the macula densa. Such contraction and relaxation regulate blood flow to the glomerulus.
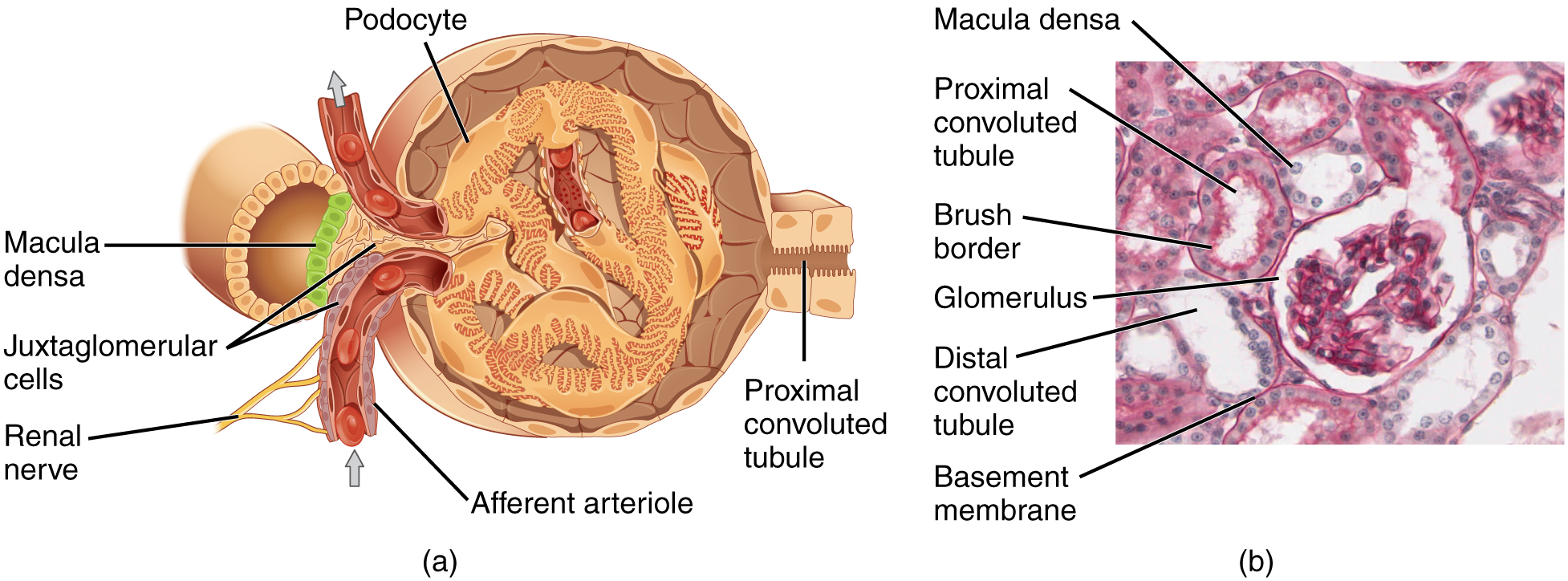
Proximal Convoluted Tubule (PCT)
Filtered fluid collected by the glomerular capsule enters into the proximal convoluted tubule (PCT). It is called convoluted due to its tortuous path. Simple cuboidal cells form this tubule with prominent microvilli on the luminal surface, forming a brush border. These microvilli create a large surface area to maximize the absorption and secretion of solutes (Na+, Cl–, glucose, etc.), the essential function of this portion of the nephron. These cells actively transport ions across their membranes, so they possess a high concentration of mitochondria to produce sufficient ATP.
Loop of Henle
The descending and ascending portions of the loop of Henle (sometimes referred to as the nephron loop) are, of course, just continuations of the same tubule. They run adjacent and parallel to each other after having made a hairpin turn at the deepest point of their descent. The descending loop of Henle consists of an initial short, thick portion and long, thin portion, whereas the ascending loop consists of an initial short, thin portion followed by a long, thick portion. The descending thick portion consists of simple cuboidal epithelium similar to that of the PCT. The descending and ascending thin portions consist of simple squamous epithelium. As you will see later, these are important differences since different portions of the loop have different permeabilities for solutes and water. The ascending thick portion consists of simple cuboidal epithelium similar to the DCT.
Distal Convoluted Tubule (DCT)
The distal convoluted tubule (DCT), like the PCT, is very tortuous and formed by simple cuboidal epithelium, but it is shorter than the PCT. These cells are not as active as those in the PCT; thus, fewer microvilli are on the apical surface. However, these cells must also pump ions against their concentration gradient, so you will find large numbers of mitochondria, although fewer than in the PCT.
Collecting Ducts
The collecting ducts are continuous with the nephron but not technically part of it. In fact, each duct collects filtrate from several nephrons for final modification via collecting tubules. Collecting tubules merge to form the collecting ducts. The collecting ducts merge as they descend deeper in the medulla to form about 30 terminal ducts, empty at a papilla. They are lined with simple squamous epithelium with receptors for ADH. When stimulated by ADH, these cells will insert aquaporin channel proteins into their membranes, which, as their name suggests, allow water to pass from the duct lumen through the cells and into the interstitial spaces to be recovered by the vasa recta. This process allows for the recovery of large amounts of water from the filtrate back into the blood. In the absence of ADH, these channels are not inserted, resulting in the excretion of water in the form of dilute urine. Most, if not all, cells of the body contain aquaporin molecules, whose channels are so small that only water can pass. At least 10 types of aquaporins are known in humans, and six are found in the kidney. The function of all aquaporins is to allow the movement of water across the lipid-rich, hydrophobic cell membrane.
Urine Formation
Urine is a fluid of variable composition that requires specialized structures to remove it from the body safely and efficiently. Blood is filtered, and the filtrate is transformed into urine at a relatively constant rate throughout the day. This processed liquid is stored until a convenient time for excretion. All structures involved in the urine’s transport and storage are large enough to be visible to the naked eye. This transport and storage system not only stores the waste, but it protects the tissues from damage due to the wide range of pH and osmolarity of the urine, prevents infection by foreign organisms, and for the male, provides reproductive functions.
The glomerular capsule and glomerulus play a critical role in filtering blood. The filtrate that leaves the glomerulus is primarily water. Thus the body has numerous mechanisms to recover the water (and electrolytes) lost during filtration.
Fetal Kidneys – Fun Fact (-> you will not be assessed on this content)
The fetal kidneys filter blood and produce urine, but the neonatal kidneys are still immature and inefficient at concentrating urine. Therefore, newborns produce very dilute urine, making it particularly important for infants to obtain sufficient fluids from breast milk or formula.
Mechanisms of Water Recovery – FYI (For Your Information -> you will not be assessed on this content)
Mechanisms by which substances move across membranes for reabsorption or secretion include active transport, diffusion, facilitated diffusion, secondary active transport, and osmosis. These were discussed in an earlier chapter, and you may wish to review them.
Active transport utilizes energy, usually the energy found in a phosphate bond of ATP, to move a substance across a membrane from a low to a high concentration. It is very specific and must have an appropriately shaped receptor for the substance to be transported. An example would be the active transport of Na+ out of a cell and K+ into a cell by the Na+/K+ pump. Both ions are moved in opposite directions from a lower to a higher concentration.
Simple diffusion moves a substance from a higher to a lower concentration down its concentration gradient. It requires no energy and only needs to be soluble.
Facilitated diffusion is similar to diffusion in that it moves a substance down its concentration gradient. The difference is that it requires specific membrane receptors or channel proteins for movement. The movement of glucose and, in certain situations, Na+ ions is an example of facilitated diffusion. In some cases of mediated transport, two different substances share the same channel protein port; these mechanisms are described by the terms symport and antiport.
Symport mechanisms move two or more substances in the same direction at the same time, whereas antiport mechanisms move two or more substances in opposite directions across the cell membrane. Both mechanisms may utilize concentration gradients maintained by ATP pumps. As described previously, when active transport powers the transport of another substance in this way, it is called “secondary active transport.” Glucose reabsorption in the kidneys is by secondary active transport. Na+/K+ ATPases on the basal membrane of a tubular cell constantly pump Na+ out of the cell, maintaining a strong electrochemical gradient for Na+ to move into the cell from the tubular lumen. On the luminal (apical) surface, a Na+/glucose symport protein assists both Na+ and glucose movement into the cell. The cotransporter moves glucose into the cell against its concentration gradient as Na+ moves down the electrochemical gradient created by the basal membranes Na+/K+ ATPases. The glucose molecule then diffuses across the basal membrane by facilitated diffusion into the interstitial space and from there into peritubular capillaries.
Most of the Ca++, Na+, glucose, and amino acids must be reabsorbed by the nephron to maintain homeostatic plasma concentrations. Other substances, such as urea, K+, ammonia (NH3), creatinine, and some drugs are secreted into the filtrate as waste products. Acid-base balance is maintained through actions of the lungs and kidneys: The lungs rid the body of H+, whereas the kidneys secrete or reabsorb H+ and HCO3–. In the case of urea, about 50 percent is passively reabsorbed by the PCT. More is recovered in the collecting ducts as needed. ADH induces the insertion of urea transporters and aquaporin channel proteins.
Substances Filtered and Reabsorbed by the Kidney per 24 Hours | |||
---|---|---|---|
Substance | Amount filtered (grams) | Amount reabsorbed (grams) | Amount in urine (grams) |
Water | 180 L | 179 L | 1 L |
Proteins | 10–20 | 10–20 | 0 |
Chlorine | 630 | 625 | 5 |
Sodium | 540 | 537 | 3 |
Bicarbonate | 300 | 299.7 | 0.3 |
Glucose | 180 | 180 | 0 |
Urea | 53 | 28 | 25 |
Potassium | 28 | 24 | 4 |
Uric acid | 8.5 | 7.7 | 0.8 |
Creatinine | 1.4 | 0 | 1.4 |
Reabsorption and Secretion in the PCT
The renal corpuscle filters the blood to create a filtrate that differs from blood mainly in the absence of cells and large proteins. From this point to the ends of the collecting ducts, the filtrate or forming urine is undergoing modification through secretion and reabsorption before true urine is produced. The first point at which the forming urine is modified is in the PCT. Here, some substances are reabsorbed, whereas others are secreted. Note the use of the term “reabsorbed.” These substances were “absorbed” in the digestive tract—99 percent of the water, and most of the solutes filtered by the nephron must be reabsorbed. Water and substances that are reabsorbed are returned to the circulation by the peritubular and vasa recta capillaries. It is important to understand the difference between the glomerulus and the peritubular and vasa recta capillaries. The glomerulus has a relatively high pressure inside its capillaries and can sustain this by dilating the afferent arteriole while constricting the efferent arteriole. This assures adequate filtration pressure even as the systemic blood pressure varies. The movement of water into the peritubular capillaries and vasa recta will be influenced primarily by osmolarity and concentration gradients. Sodium is actively pumped out of the PCT into the interstitial spaces between cells and diffuses down its concentration gradient into the peritubular capillary. As it does so, water will follow passively to maintain an isotonic fluid environment inside the capillary. This is called obligatory water reabsorption because water is “obliged” to follow the Na+.
More substances move across the membranes of the PCT than any other portion of the nephron. About 67 percent of the water, Na+, and K+ entering the nephron is reabsorbed in the PCT and returned to circulation. Almost 100 percent of glucose, amino acids, and other organic substances such as vitamins are normally recovered. The significant recovery of solutes from the PCT lumen to the interstitial space creates an osmotic gradient that promotes water recovery.
Reabsorption and Secretion in the Loop of Henle
The loop of Henle consists of two sections: thick and thin descending and thin and thick ascending sections. The loops of cortical nephrons do not extend into the renal medulla very far, if at all. Juxtamedullary nephrons have loops that extend variable distances, some very deep into the medulla. The descending and ascending portions of the loop are highly specialized to enable recovery of much of the Na+ and water filtered by the glomerulus.
Descending Loop
The majority of the descending loop is comprised of simple squamous epithelial cells; to simplify the function of the loop, this discussion focuses on these cells. These membranes allow unrestricted movement of water from the descending loop into the surrounding interstitium. This increase results in the reabsorption of up to 15 percent of the water entering the nephron. Modest amounts of urea, Na+, and other ions are also recovered here.
Most of the solutes that were filtered in the glomerulus have now been recovered along with a majority of water, about 82 percent. As the forming urine enters the ascending loop, major adjustments will be made to the concentration of solutes to create what you perceive as urine.
Ascending Loop
The ascending loop is made of very short thin, and longer thick portions. Once again, to simplify the function, this section only considers the thick portion. The thick portion is lined with simple cuboidal epithelium. Mainly Na+ and Cl– are actively reabsorbed in this portion. This has two significant effects: Removal of NaCl while retaining water leads to a hypoosmotic filtrate by the time it reaches the DCT; pumping NaCl into the interstitial space contributes to the hyperosmotic environment in the kidney medulla.
Reabsorption and Secretion in the Distal Convoluted Tubule
Approximately 80 percent of filtered water has been recovered by the time the dilute forming urine enters the DCT. Cells of the DCT also recover Ca++ from the filtrate. The DCT will recover another 10–15 percent before the forming urine enters the collecting ducts. Peritubular capillaries receive the solutes and water, returning them to the circulation.
Ureters, Bladder, Urethra, and Micturation
Once the blood flowing through the kidneys is filtered and resorbed, the filtrate, now called urine, must exit the body. From the collecting tubules and ducts, urine is emptied into minor calyxes, major calyxes, and ultimately the renal pelvis before entering the ureters.
Ureters
The kidneys and ureters are completely retroperitoneal, and the bladder has a peritoneal covering only over the dome. As urine is formed, it drains into the calyces of the kidney, which merge to form the funnel-shaped renal pelvis in the hilum of each kidney. The renal pelvis narrows to become the ureter of each kidney. As urine passes through the ureter, it does not passively drain into the bladder but rather is propelled by waves of peristalsis. As the ureters enter the pelvis, they sweep laterally, hugging the pelvic walls. As they approach the bladder, they turn medially and pierce the bladder wall obliquely. This is important because it creates a one-way valve (a physiological sphincter rather than an anatomical sphincter) that allows urine into the bladder but prevents reflux of urine from the bladder back into the ureter. Children born lacking this oblique course of the ureter through the bladder wall are susceptible to “vesicoureteral reflux,” which dramatically increases their risk of serious UTI. Pregnancy also increases the likelihood of reflux and UTI.
The ureters are approximately 30 cm long. The inner mucosa is lined with transitional epithelium and scattered goblet cells that secrete protective mucus. The muscular layer of the ureter consists of longitudinal and circular smooth muscles that create the peristaltic contractions to move the urine into the bladder without the aid of gravity. Finally, a loose adventitial layer composed of collagen and fat anchors the ureters between the parietal peritoneum and the posterior abdominal wall.
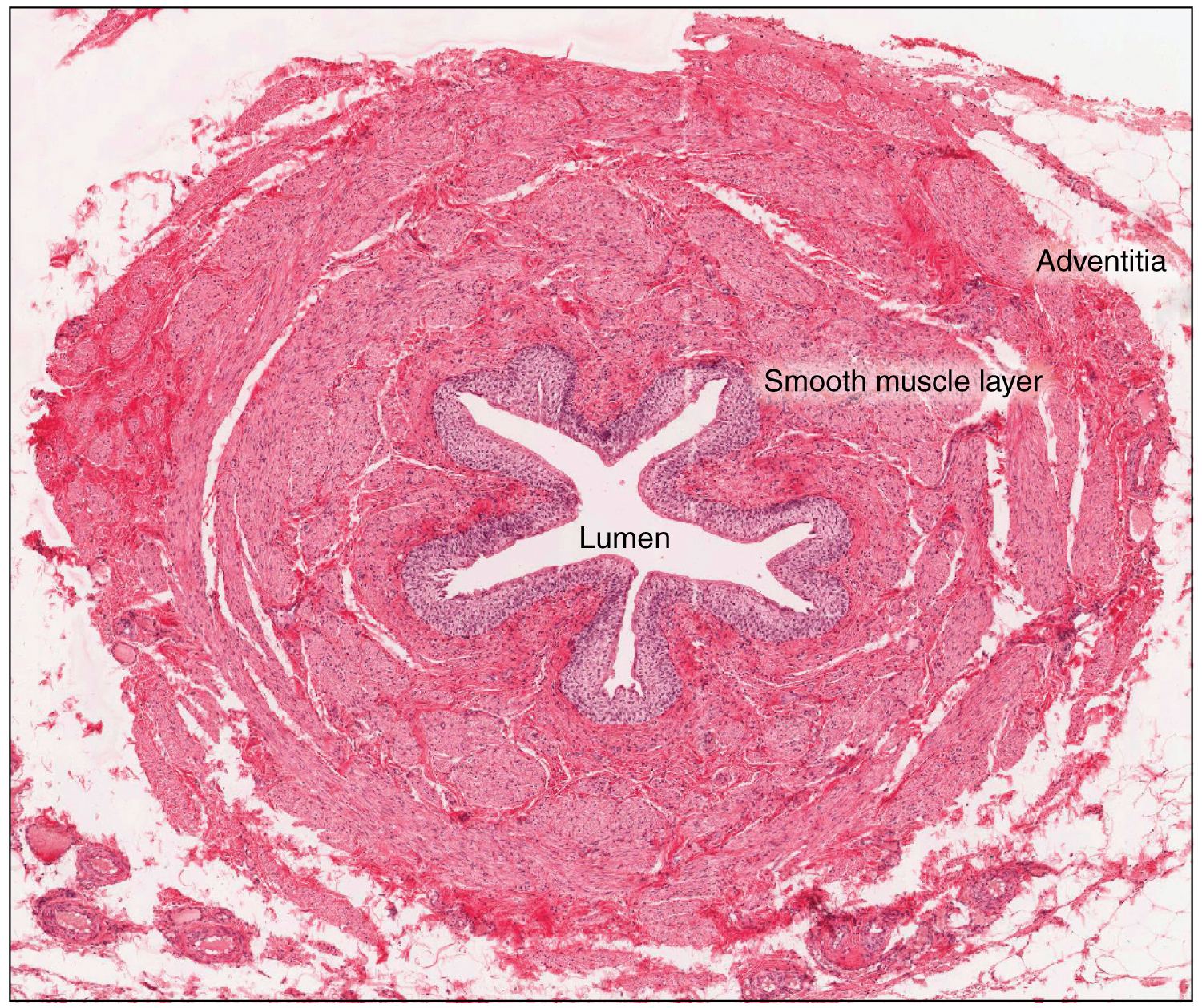
Bladder
The urinary bladder collects urine from both ureters. The bladder lies anterior to the uterus in females, posterior to the pubic bone, and anterior to the rectum. During late pregnancy, its capacity is reduced due to compression by the enlarging uterus, resulting in increased urination frequency. In males, the anatomy is similar, minus the uterus, and with the addition of the prostate inferior to the bladder. The bladder is partially retroperitoneal (outside the peritoneal cavity), with its peritoneal-covered “dome” projecting into the abdomen when the bladder is distended with urine.
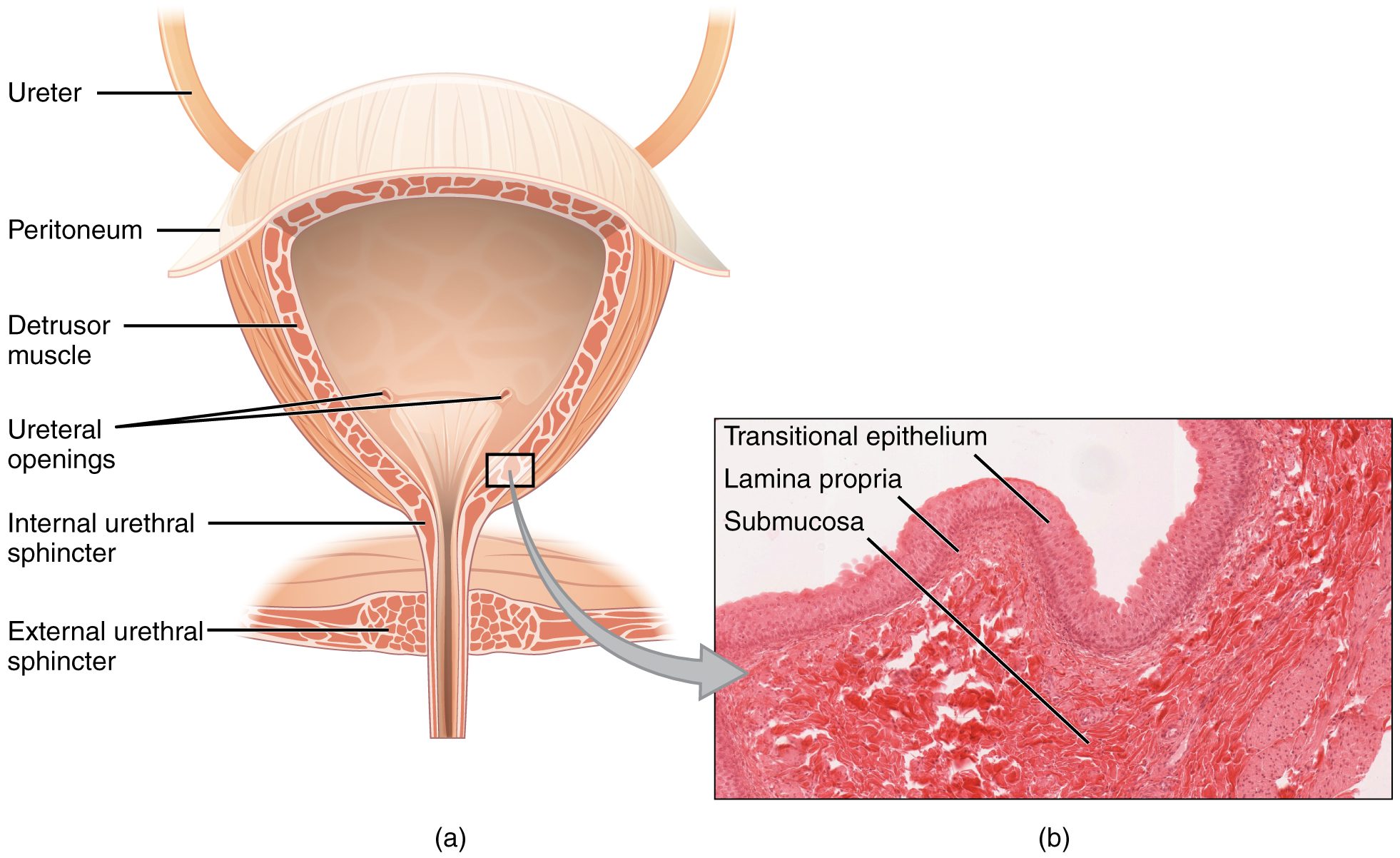
The detrusor muscle contracts with significant force in the young. The bladder’s strength diminishes with age, but voluntary contractions of abdominal skeletal muscles can increase intra-abdominal pressure to promote more forceful bladder emptying. Such voluntary contraction is also used in forceful defecation and childbirth.
Urethra
The urethra transports urine from the bladder to the outside of the body for disposal. The urethra is the only urologic organ that shows any significant anatomic difference between males and females; all other urine transport structures are identical.
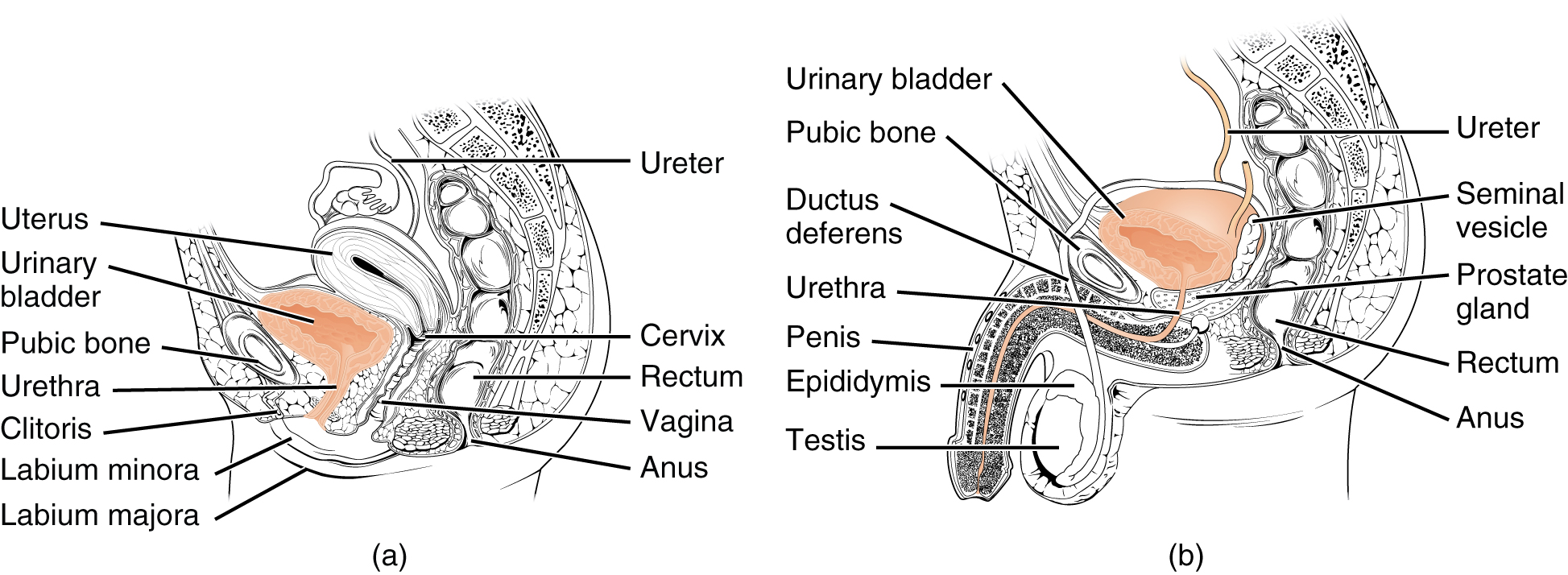
The urethra in both males and females begins inferior and central to the two ureteral openings forming the three points of a triangular-shaped area at the base of the bladder called the trigone (Greek tri- = “triangle” and the root of the word “trigonometry”). The urethra tracks posterior and inferior to the pubic symphysis. In both males and females, the proximal urethra is lined by transitional epithelium, whereas the terminal portion is a nonkeratinized, stratified squamous epithelium. In the male, pseudostratified columnar epithelium lines the urethra between these two cell types. Voiding is regulated by an involuntary autonomic nervous system-controlled internal urinary sphincter, consisting of smooth muscle and voluntary skeletal muscle that forms the external urinary sphincter below it.
Female Urethra
The external urethral orifice is embedded in the anterior vaginal wall inferior to the clitoris, superior to the vaginal opening, and medial to the labia minora. Its short length, about 4 cm, is less of a barrier to fecal bacteria than the longer male urethra and the best explanation for the greater incidence of UTI in women. Voluntary control of the external urethral sphincter is a function of the pudendal nerve. It arises in the spinal cord’s sacral region, traveling via the S2–S4 nerves of the sacral plexus.
Male Urethra
The male urethra passes through the prostate gland immediately inferior to the bladder before passing below the pubic symphysis. The length of the male urethra varies between men but averages 20 cm in length. It is divided into three regions: the prostatic urethra, the membranous urethra, and the spongy urethra. The prostatic urethra passes through the prostate gland. During sexual intercourse, it receives sperm via the ejaculatory ducts and secretions from the seminal vesicles. Paired bulbourethral (Cowper’s) glands produce and secrete mucus into the urethra to buffer urethral pH during sexual stimulation. The mucus neutralizes the usually acidic environment and lubricates the urethra, decreasing the resistance to ejaculation. The membranous urethra passes through the deep muscles of the perineum, where it is invested by the overlying urethral sphincters. The spongy urethra exits at the tip (external urethral orifice) of the penis after passing through the corpus spongiosum. Mucous glands are found along much of the length of the urethra and protect the urethra from extremes of urine pH. Innervation is the same in both males and females.
Micturition Reflex
Micturition is a less-often used but the proper term for urination or voiding. It results from an interplay of involuntary and voluntary actions by the internal and external urethral sphincters. When bladder volume reaches about 150 mL, an urge to void is sensed but is easily overridden. Voluntary control of urination relies on consciously preventing the external urethral sphincter’s relaxation to maintain urinary continence. As the bladder fills, subsequent urges become harder to ignore. Ultimately, voluntary constraint fails with resulting incontinence, which will occur as bladder volume approaches 300 to 400 mL.
Normal micturition is a result of stretch receptors in the bladder wall that transmit nerve impulses to the sacral region of the spinal cord to generate a spinal reflex. The resulting parasympathetic neural outflow causes contraction of the detrusor muscle and relaxation of the involuntary internal urethral sphincter. At the same time, the spinal cord inhibits somatic motor neurons, resulting in the relaxation of the skeletal muscle of the external urethral sphincter. The micturition reflex is active in infants, but with maturity, children learn to override the reflex by asserting external sphincter control, thereby delaying voiding (potty training). This reflex may be preserved even in the face of spinal cord injury that results in paraplegia or quadriplegia. However, relaxation of the external sphincter may not be possible in all cases, and therefore, periodic catheterization may be necessary for bladder emptying.
Nerves involved in the control of urination include the hypogastric, pelvic, and pudendal. Voluntary micturition requires an intact spinal cord and functional pudendal nerve arising from the sacral micturition center. Since the external urinary sphincter is voluntary skeletal muscle, actions by cholinergic neurons maintain contraction (and thereby continence) during filling of the bladder. At the same time, sympathetic nervous activity via the hypogastric nerves suppresses contraction of the detrusor muscle. With further bladder stretch, afferent signals traveling over sacral pelvic nerves activate parasympathetic neurons. This activates efferent neurons to release acetylcholine at the neuromuscular junctions, producing detrusor contraction and bladder emptying.