Module 2: Tissues II – Muscle and Nervous
Learning Objectives:
By the end of this class, students will be able to:
- Compare and contrast skeletal, cardiac, and smooth muscle.
- Describe the properties, and functions of skeletal muscle tissue.
- Describe the anatomy of skeletal muscle tissue.
- Analyze how the size of a motor unit contributes to the function of a muscle.
- Summarize the effects of exercise and aging on muscle tissue.
- Describe the divisions of the nervous system and their contents.
- Understand how neurons communicate with each other.
- Compare and contrast the nervous system and endocrine system.
- Identify the different types of neurons and glial cells and their functions.
- Understand the process of axonal regeneration and apply that knowledge to nervous system injury and pathology.
- Describe the different types neural modalities.
Terms to Know
Types of Muscle Tissue
Skeletal Muscle Tissue – Details
*Covered only in lecture, not in this text |
Nervous System
Neuron
Glial Cells
Nerves
|
Muscle Tissue
This content is covered in the assignment and reviewed and built upon in lecture.
Muscle is one of the four primary tissue types of the body. All types of muscle tissues function to produce some form of movement. This includes movements of our bones, joints, and skin (muscles of facial expression); movement of our heart as it contracts; movements in our internal structures, such as our digestive tract, blood vessels, and iris of our eye; and movements that open and close body passageways.
All muscle cells, or myocytes, share the following characteristics:
- Excitability: can respond to a stimulus.
- Contractility: can contract and shorten the length of the fiber. The contraction of muscle tissue can be under voluntary, or conscious control, or involuntary, unconscious control.
- Extensibility: can stretch or extend beyond its resting length.
- Elasticity: can return to its original length when relaxed.
Types of Muscle Tissue
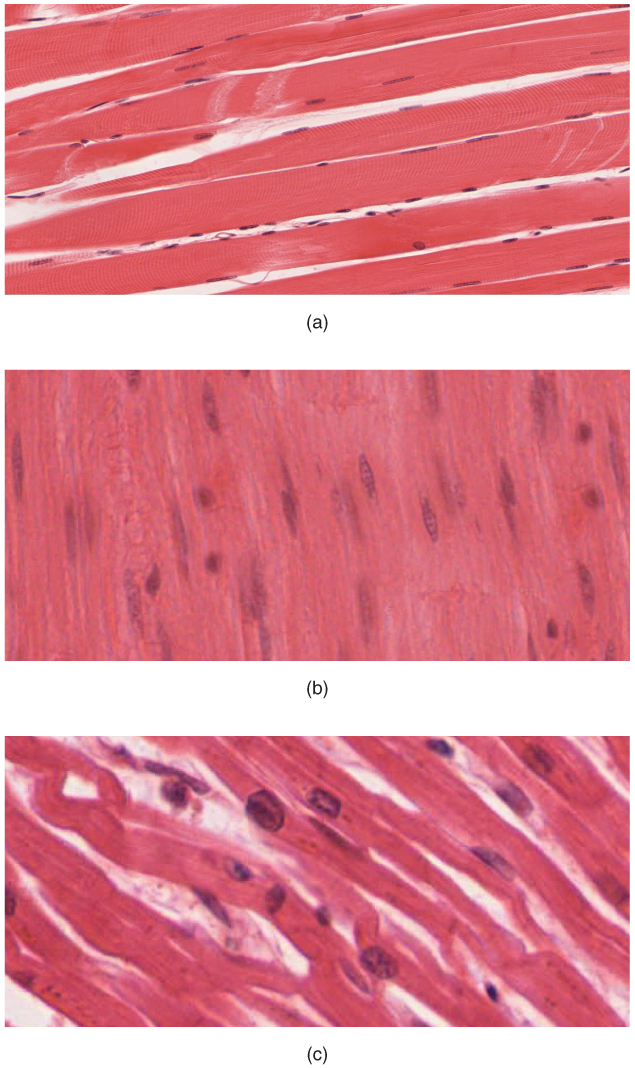
This information is covered in the assignment and built upon in lecture.
Muscle tissue is classified into three types according to structure and function: skeletal, cardiac, and smooth. Skeletal muscle tissue forms the muscles that move our bones and joints. Skeletal muscle fibers, or muscle cells, are long, cylindrical fibers that span the entire length of a muscle. We will discuss skeletal muscle more in-depth later in this module. Cardiac muscle tissue is found in the heart. We will discuss the heart and cardiac muscle more in module 13. Cardiomyocytes, or cardiac muscle fibers, are much smaller and shorter than skeletal muscle fibers, and they are extensively branched. They contain intercalated discs with gap junctions that form communication channels between adjacent cardiomyocytes, allowing cardiac muscle cells to contract in a wave-like pattern so the heart can work as a coordinated pump. Both skeletal and cardiac muscle tissue appears striated, or striped, due to the arrangement of their contractile proteins. Smooth muscle is so-named because the cells do not have striations. They are thousands of times shorter than skeletal muscle fibers. Unlike other muscle tissue, smooth muscle tissue can also divide to produce more cells, a process called hyperplasia.
Skeletal muscle completely depends on innervation from the nervous system to contract. Cardiac muscle and smooth muscle can respond to electrical stimuli from the nervous system as well as hormones and local stimuli. In certain locations, such as the walls of visceral organs, stretching the muscle can trigger smooth muscle contraction. Because most smooth muscles must function for long periods without rest, their power output is relatively low, but contractions can continue without using large amounts of energy.
Compare and contrast the features of the different types of muscle tissues:
Cardiac Muscle | Smooth Muscle | Skeletal Muscle | |
Myocyte Shape | Small, Short, branched | Small, spindle-shaped (wide in the middle & tapered on each end, somewhat like a football) | Large, long, cylindrical |
Striated? | Yes | No | Yes |
Voluntary or Involuntary? | Involuntary | Involuntary | Voluntary |
Location | Heart wall | Walls of many internal organs and passageways (digestive, respiratory, blood vessels, etc.), eye, skin, and more | Muscles acting on the limbs & trunk, muscles of facial expression, external sphincters regulating passage out of the body |
Function | Circulate blood | Move food, urine, reproductive secretions through their respective systems Control the diameter of blood vessels, respiratory tracts, iris |
Movement or stabilization of the skeleton, guard entrances and/or exits for the digestive, respiratory, and urinary systems Heat production Protects internal organs |
Connected by intercalated discs with gap junctions | Yes | No | No |
Capable of cell division to produce more muscle cells | No | Yes | No |
More About Skeletal Muscle
This information is covered in the assignment and reviewed and built upon in lecture.
Skeletal muscle fibers can be quite large, spanning the entire length of the muscle. For example, the individual skeletal muscle fibers in your rectus femoris muscle, one of the quadriceps muscles of your anterior thigh, spans the distance of the muscle running from your anterior hip to your knee.
The number of skeletal muscle fibers is set during development and stays relatively constant throughout life, as skeletal muscle fibers cannot divide to produce more fibers. However, skeletal muscles are still capable of repair due to the presence of special cells called satellite cells (more on that in lecture).
Skeletal muscle produces movement through voluntary contraction, such as movement of our limbs, trunk, external sphincters, and muscles of facial expression. In addition to producing movement, skeletal muscle has a few other key functions:
- Maintain posture and stabilize joints: Contraction allows us to maintain our posture, resist gravity, and adds dynamic stability to our joints (vs. static stability that comes from ligaments, for example). This contraction is occurring even though the muscle isn’t actively shortening.
- Generate heat: Skeletal muscle generates heat as a byproduct of its contraction. Shivering is an involuntary contraction of skeletal muscles in response to perceived lower than normal body temperature.
- Protect internal organs: Forms a physical barrier between our internal organs and the outside world (i.e. the muscles of our trunk).
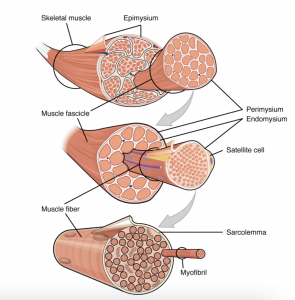
Each skeletal muscle is an organ that consists of skeletal muscle fibers, blood vessels, nerve fibers, and connective tissue. Inside each skeletal muscle, muscle fibers are organized into individual bundles, called fascicles. Each skeletal muscle has three layers of connective tissue that enclose it, provide structure and support to the muscle as a whole, and compartmentalize the muscle fibers within the muscle.
- Epimysium: a sheath of dense, irregular connective tissue that surrounds each muscle
- Perimysium: a sheath of connective tissue surrounding each individual fascicle
- Endomysium: a sheath of connective tissue surrounding each individual muscle fiber
Motor Units
This information is covered in lecture.
Every skeletal muscle fiber must be innervated by a motor neuron in order to contract. The neuromuscular junction is the site where a motor neuron meets the muscle fiber. Each muscle fiber is innervated by only one motor neuron, but one motor neuron can innervate several skeletal muscle fibers. The group of muscle fibers in a muscle innervated by a single motor neuron is called a motor unit.
The size of a motor unit is variable depending on the nature of the muscle.
- Small motor unit = one motor neuron supplies a small number of fibers in a muscle
- Small motor units permit very fine motor control of the muscle.
- Examples: muscles that move the eye (extraocular muscles) or muscles of the hand, where a single motor neuron can supply less than ten muscle fibers
- Large motor unit = one motor neuron supplies a large number of muscle fibers in a muscle
- Large motor units are concerned with simple, or “gross” (large), movements.
- Examples: thigh muscles or back muscles, where a single motor neuron will supply thousands of muscle fibers
When a motor neuron is stimulated, all muscle fibers in its motor unit will contract. This is the all-or-none principle. The number of and specific motor units in a muscle recruited to contract at a given time will vary depending on the motion and strength required to complete an action. As more motor units are recruited and contract, the muscle contraction grows progressively stronger. When necessary, the maximal number of motor units in a muscle can be recruited simultaneously, producing the maximum force of contraction for that muscle, but this cannot last for very long because of the energy requirements to sustain the contraction. To prevent complete muscle fatigue, motor units are generally not all simultaneously active, but instead some motor units rest while others are active, which allows for longer and more efficient muscle contractions.
Types of Skeletal Muscle Fibers
This information is covered in lecture.
There are three main types of muscle fibers, as classified by the speed of their contractions and the fuel they use to produce energy.
- Slow oxidative (SO) fibers: These fibers contract relatively slowly. They are aerobic, meaning they primarily use oxygen in the energy-producing process. As a result, they need a greater blood supply to bring oxygen to them. These fibers produce less power, but have greater endurance, meaning they can contract for longer periods of time than other types of fibers. The ability to function for long periods without fatiguing makes them useful in maintaining posture, producing isometric contractions, stabilizing bones and joints, and making small movements that happen often but do not require large amounts of energy. As a result, they are the predominant fiber type used in endurance (aerobic) exercise.
- Fast oxidative (FO) fibers: (We won’t ask you about the details of these fibers on the exam, but here is a brief description of them in case you are interested). These are often called intermediate fibers. They have relatively fast contractions, and they fatigue more quickly than slow oxidative fibers. They primarily use oxygen to produce energy, but they can switch to using glucose (anaerobic). These fibers are used primarily for movements, such as walking, that require more energy than postural control but less energy than an explosive movement, such as sprinting.
- Fast glycolytic (FG) fibers: These fibers have fast contractions and primarily use glucose in the energy-producing process, which is located within the muscle. Fast glycolytic fibers are used to produce rapid, forceful contractions to make quick, powerful movements. However, they fatigue more quickly than the other fiber types.
Most skeletal muscles contain a mixture of all three types. The predominant fiber type in a muscle is determined by the primary function of the muscle. The proportion of slow muscle fibers in muscle determines the suitability of that muscle for endurance activities. Resistance exercises require large amounts of fast glycolytic fibers to produce short, powerful movements that are not repeated over long periods of time. Muscles used for power movements have a higher ratio of fast glycolytic fibers to slow oxidative fibers.
Physical exercise alters the appearance of skeletal muscles and can produce changes in muscle performance. Endurance training modifies several features of slow oxidative fibers to make them even more efficient. Resistance exercise leads to an increased number of myofibrils in the muscle fibers. This results in an increased size of the muscle cells, or hypertrophy, and ultimately an increased size of the muscle. However, exercise does NOT result in the formation of new muscle fibers. Conversely, a lack of use can result in a decrease in muscle mass, called atrophy.
Nervous Tissue
Nervous tissue, and the nervous system as a whole, transmits and receives electrochemical signals that provide the body with information. The nervous system can be divided into two major regions: the central and peripheral nervous systems. The central nervous system (CNS) consists of the brain and spinal cord. The peripheral nervous system (PNS) consists of all nervous tissue outside of the brain and spinal cord.
In both the CNS and PNS two main classes of cells make up nervous tissue: neurons and neuroglia. Neurons process and communicate information throughout the body. Glial cells, or neuroglia, play an essential role in supporting neurons.
Neurons
This information is covered in the assignment and reviewed and built upon in lecture.
Neurons, or nerve cells, transmit information through the body via electrochemical signals. There are many neurons in the nervous system—a number in the trillions. The synapse is the gap between two neurons, or between a neuron and its target, a muscle or a gland, across which the impulse is transmitted by chemical compounds known as neurotransmitters.
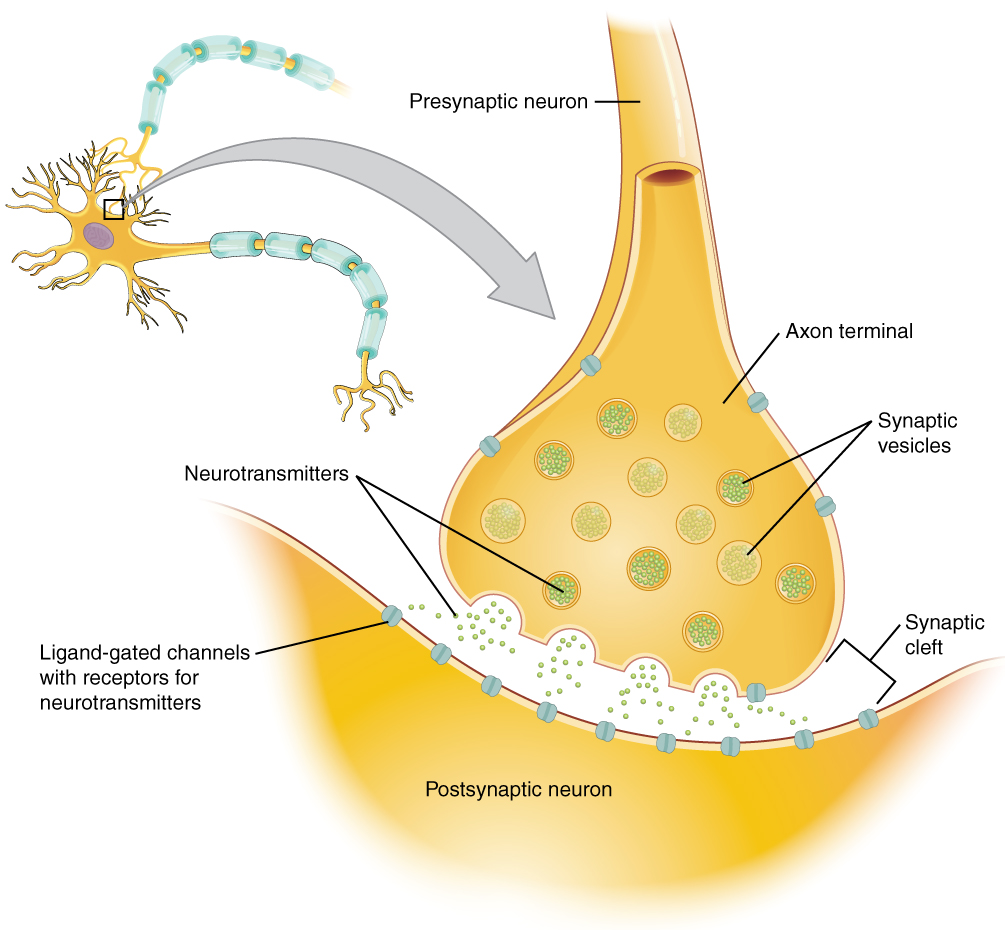
The structure of neurons is well-suited to their function as conducting cells, with three main parts:
- Cell body (soma): The cell body includes most of the cytoplasm, the organelles, and the nucleus. It is the processing or “thinking” part of the neuron.
- Dendrite: These branches receive most of the input from other neurons and carry it to the cell body. The dendrites are usually highly branched processes, providing locations for other neurons to communicate with the cell body.
- Axon: Neurons typically have one, and only one, axon—a fiber that emerges from the cell body and projects to target cells. It is the axon that propagates the nerve impulse, which is communicated to one or more cells. The single axon can branch repeatedly to communicate with many target cells. That target could be another neuron, a muscle fiber, or a gland. Where the axon emerges from the cell body, there is a special region referred to as the axon hillock. This is a tapering of the cell body toward the axon fiber.
Information flows through a neuron from the dendrites, across the cell body, and down the axon. This gives the neuron a polarity—meaning that information flows in this one direction.
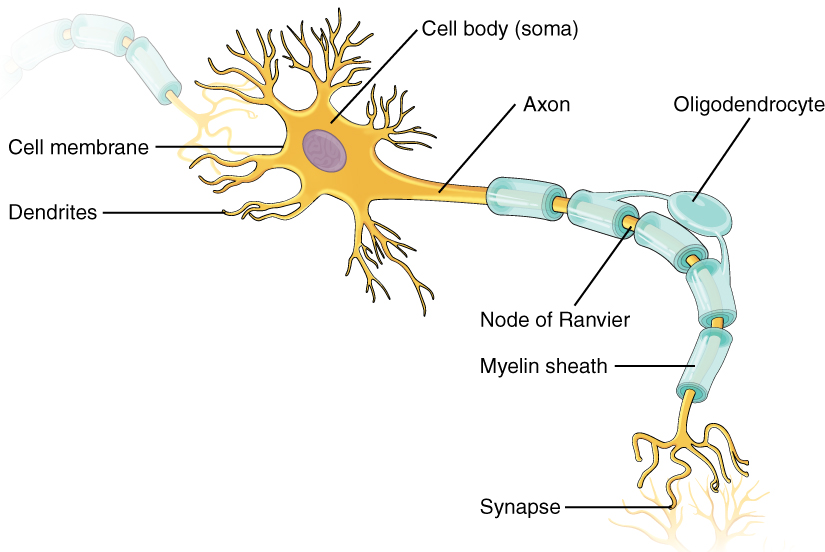
Neurons can be categorized by shape:
- Pseudounipolar neurons: True unipolar cells are only found in invertebrate animals, so the unipolar cells in humans are more appropriately called “pseudo-unipolar” cells. Pseudounipolar cells have an axon that emerges from the cell body, but it splits so that the axon can extend along a very long distance. At one end of the axon are dendrites, and at the other end, the axon forms synaptic connections with a target. Pseudounipolar cells are exclusively sensory neurons and have two unique characteristics.
- Bipolar neurons: These neurons have two processes, which extend from each end of the cell body, opposite to each other. One is the axon and one the dendrite. Bipolar cells are not very common. They are found mainly in the olfactory epithelium (where smell stimuli are sensed), and as part of the retina.
- Multipolar neurons: Multipolar neurons are all of the neurons that are not unipolar or bipolar. They are by far the most common neurons in the body. They have one axon and two or more dendrites (usually many more).
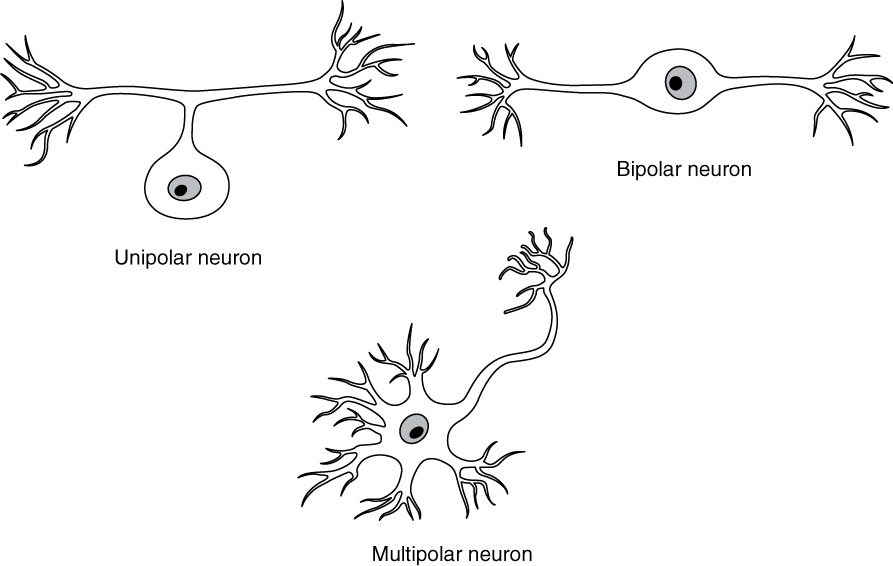
Glial Cells
This information is covered in the assignment and reviewed and built upon in lecture.
Glial cells, or neuroglia, are cells that support neurons. Many glial functions are directed at helping neurons complete their function of communication. There are six different glial cells, with four found in the CNS and two found in the PNS.
Glial Cells of the Central Nervous System:
- Astrocytes: Astrocytes are the most abundant glial cells in the CNS. Named for their star shape, they have many processes extending from their main cell body. Those processes extend to interact with neurons and blood vessels. Astrocytes have many functions, most of which serve to support neurons, including:
- Regulate the environment around neurons and takes up and/or breaks down some neurotransmitters or ions in the fluid surrounding the neurons.
- Contribute to the blood-brain barrier, a physiological barrier that keeps many substances that circulate in the rest of the body from getting into the central nervous system, restricting what can cross from circulating blood into the CNS. Certain molecules, such as glucose, can pass through, but other molecules cannot.
- Act like the “connective tissue of the brain, filling spaces and holding things together
- Form scar tissue of the brain by proliferating and surrounding damaged regions to separate it from the healthy neurons. This protects healthy neurons from chemical cascade that occurs as a result of the damage.
- Regulate the inflammatory response to damage.
- Involved in synapse formation and neuronal growth in developing nervous tissue.
- Propagate calcium signals involved with memory.
- Microglia: These glial cells are the smallest and least-abundant in the CNS. They can be considered the immune cells of the brain. White blood cells, which are a primary line of defense in the body, cannot get through the blood brain barrier. Instead, microglia are constantly patrolling the CNS, extending and retracting their processes to inspect the brain and spinal cord tissue. If there is damage to nervous tissue in the CNS, chemicals are sensed by the microglia and draw them to the injury site. The microglia then phagocytize debris from the dead or dying cells and invading microorganisms.
- Oligodendrocytes: These glial cells myelinate axons in the central nervous system. They contain a few processes (far fewer than astrocytes), each extending out from the cell body to myelinate a portion of an axon. One oligodendrocyte will provide the myelin for multiple axon segments, either for the same axon or for separate axons. Myelination will be discussed more later in this module.
- Ependymal cells: These glial cells filter blood to make cerebrospinal fluid (CSF), the fluid that circulates through the CNS. They line each ventricle, the open spaces in the brain, and the cilia on their surface help to move CSF through the CNS.
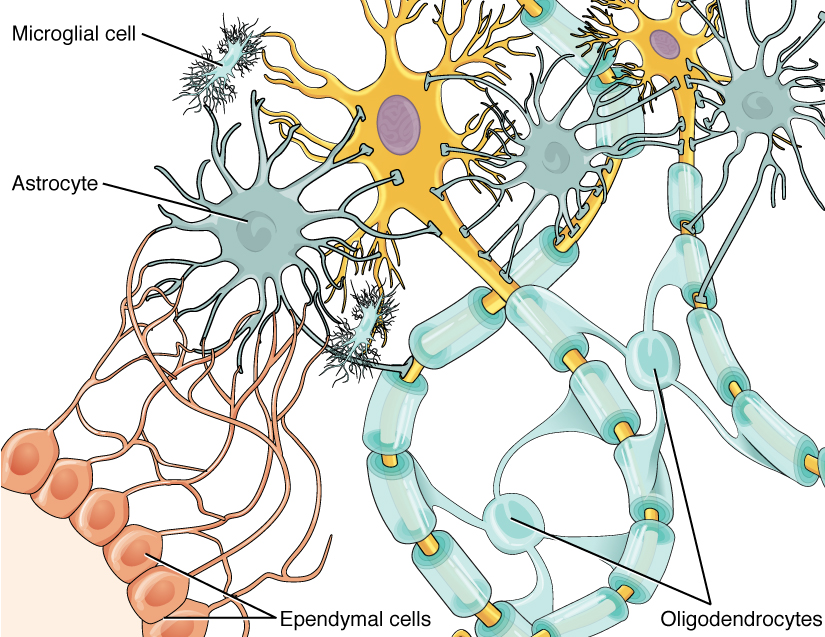
Glial Cells of the Peripheral Nervous System
- Schwann cells: They myelinate axons in the periphery. Schwann cells are different than oligodendrocytes, in that a Schwann cell wraps its entire self around one part of only one axon segment.
- Satellite cells: These glial cells surround the cell bodies of neurons in the PNS, isolating them and protecting them from the surrounding tissue. They provide support, performing similar functions in the periphery as astrocytes do in the CNS.
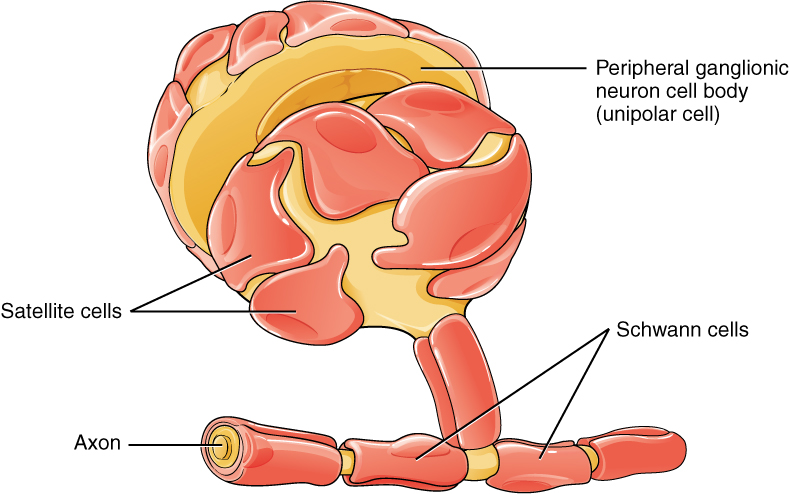
Myelination
This information is covered in lecture.
Myelin is a lipid-rich sheath that surrounds the axon and creates a myelin sheath that facilitates the transmission of electrical signals along the axon. Myelin acts as insulation much like the plastic or rubber that is used to insulate electrical wires. There are gaps in the myelin covering of an axon. Each gap is called a node of Ranvier and assists with the speed of conduction along an axon.
Myelination occurs when all or a portion of a glial cell wraps around the axon many times with little or no cytoplasm between the layers. For oligodendrocytes in the CNS, the rest of the cell is separate from the process that forms a myelin sheath around a given axon segment. For Schwann cells in the PNS, the entire cell wraps itself around the axon.
The process of myelination occurs throughout development, even into one’s 20s or 30s in some brain pathways. Some myelin sheaths are relatively thin, while others can be very thick, many times thicker than the diameter of the axon it is myelinating. The thicker the myelin, the faster the signal travels along the axon.
Not all axons are myelinated, and unmyelinated axons have the slowest conduction speed. Unmyelinated axons in the PNS are still surrounded by Schwann cells, but they are not myelinated, meaning the Schwann cells do not wrap themselves in layers around unmyelinated cells. Instead, one Schwann cell can engulf and surround a portion of several nearby axons. This is explained in more detail in lecture.
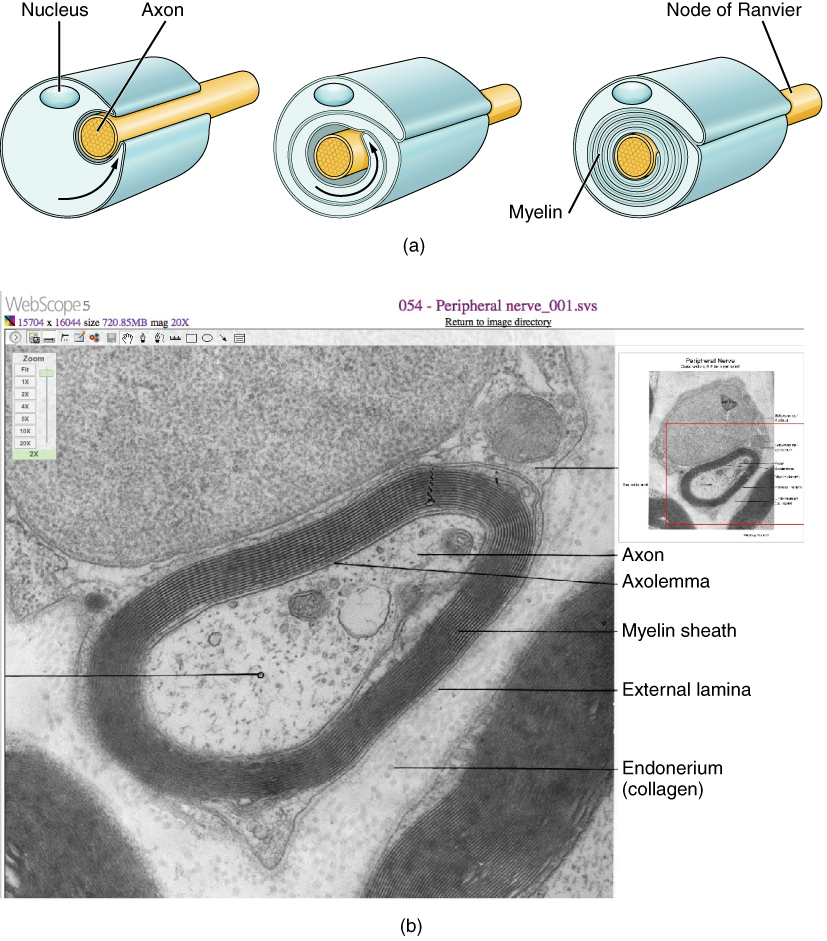
Terminology: Collections of Cell Bodies or Axons
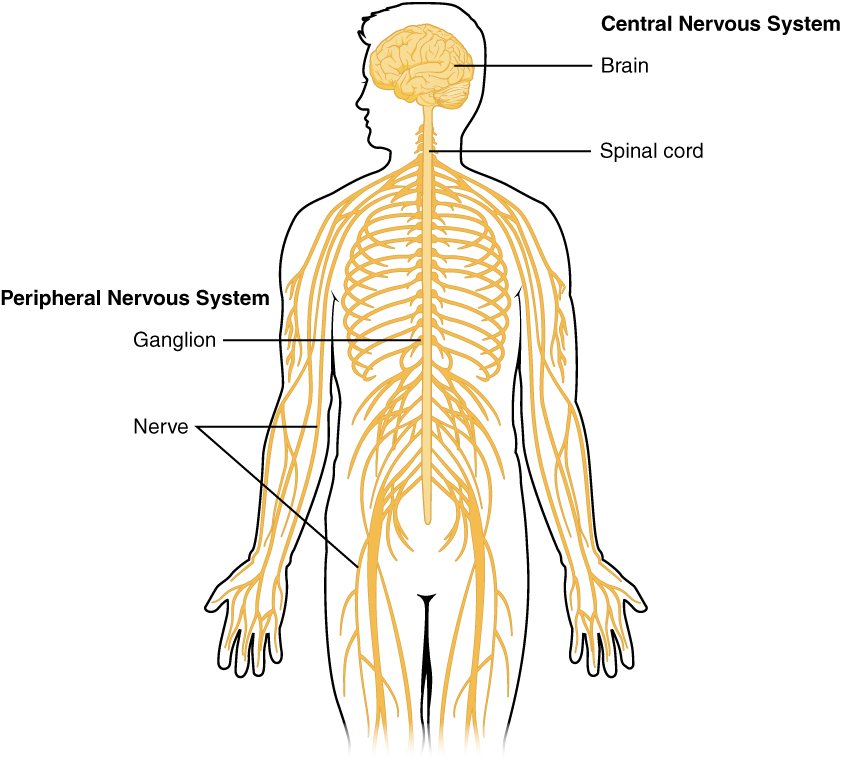
This information is covered in the assignment and reviewed and built upon in lecture.
Collections of neuronal cell bodies or axons have names that differ depending on whether the structure is in the CNS or PNS.
- A group of neuronal cell bodies:
- CNS = nucleus
- PNS = ganglion (ganglia is plural)
- Bundle of axons (fibers)
- CNS = tract (most common), also fascicle, lemniscus, commissure
- PNS = nerve
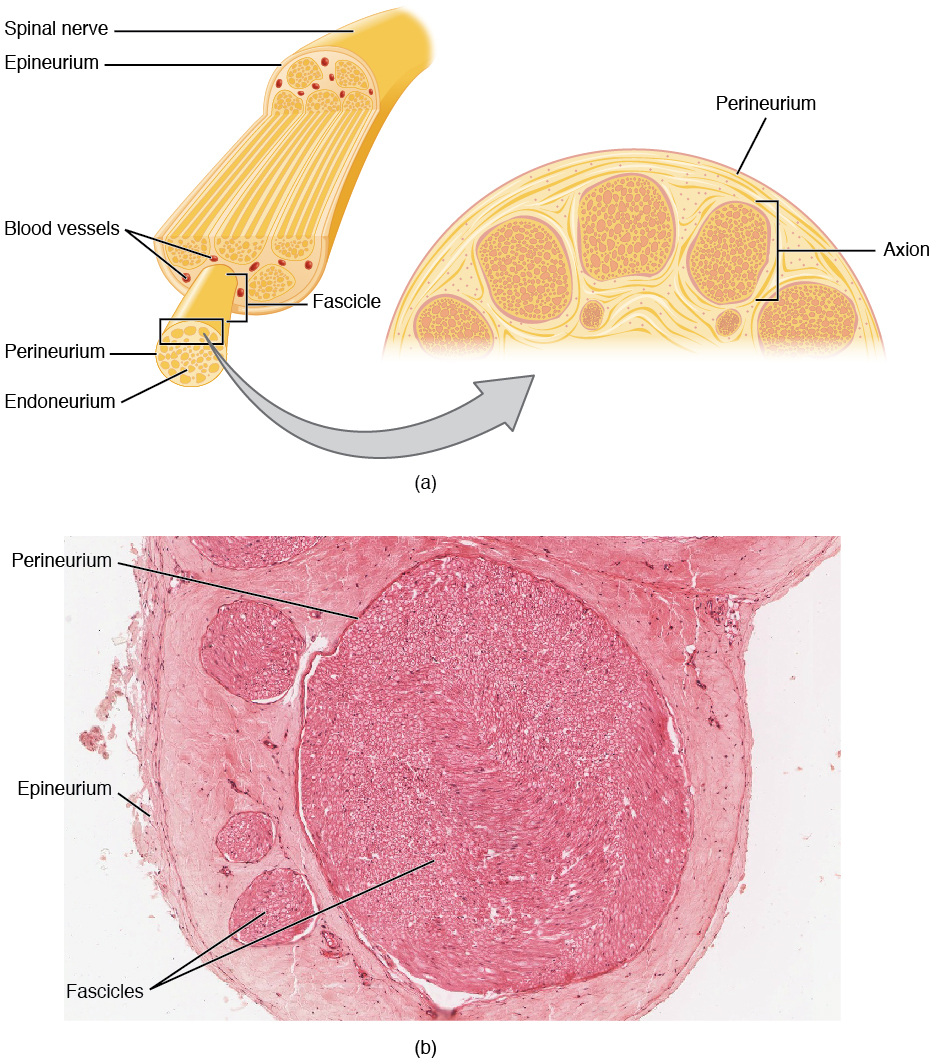
Functional Classifications in the Nervous System
This information is covered in lecture.
The nervous system is involved in receiving information about the environment around us (sensation) and generating responses to that information (motor responses). Sensory information travels from the periphery to the CNS via a sensory neuron. We sometimes call fibers that carry sensory information afferent fibers. That information is then integrated and processed in order to decide how to respond to that information. That integration can happen in the spinal cord, as with reflexes, for example. Most often that integration happens in the brain and involves tying together past experiences with a variety of sensory information to decide on a response. Ultimately the response occurs as motor output via a motor neuron that originates in the CNS and travels to either a muscle or gland. We sometimes call fibers that carry outgoing motor information efferent fibers.
Neural Modalities
This information is covered in lecture.
Neural modalities are classifications of nervous tissue functions. There are six neural modalities we will discuss in this course, but the two “special” modalities only apply to the head and neck. We will discuss those “special” modalities in unit 3. (One of the special modalities will be combined with a somatic modality, so we will really only categorize five neural modalities in this course.)
The non-special modalities are termed “general” modalities. The four general modalities are categorized in two ways. One categorization is by whether the nerve fibers are carrying sensory or motor information. The other is based on whether or not the nerve fibers are carrying somatic or visceral information. The somatic nervous system is responsible for our conscious perception of the environment and for our voluntary responses to that perception by means of skeletal muscles. Visceral information involves unconscious sensory and motor activity. Visceral motor activity is part of the autonomic nervous system, which will be covered in Unit 2.
As a result, we can categorize general nervous system fibers based on four general functional modalities:
- General somatic sensory: our sense of touch, pressure, pain, temperature, and proprioception
- General somatic motor: voluntary movement by skeletal muscles
- General visceral motor: involuntary movement of our cardiac and smooth muscle and secretion from glands
- General visceral sensory: monitoring and sensing unconscious information primarily coming from our organs and glands