Module 22: Hearing and Vestibulation
Learning Objectives:
By the end of this class, students will be able to:
- List the regions of the ear and explain the function of each region.
- List the anatomical structures in each region of the ear and define their functions.
- Compare and contrast the bony labyrinth, membranous labyrinth, and the structures found in each.
- Explain the differences or similarities between the cochlear duct and the vestibular complex.
- Describe how sound is sensed and processed, using the anatomical structures found in the cochlea.
- Explain how equilibrium sensations are sensed and processed using the anatomical structures of the vestibular complex.
- Differentiate how the body senses acceleration/linear movement vs. rotational movement.
- Describe the pathways vestibular and auditory senses take to reach their processing centers in the brain.
Terms to Know
Ear Anatomy
|
Hearing
Vestibulation
|
Anatomy of the Ear
This content will be covered in the assignment and will be only briefly reviewed in lecture.
The ear can be divided into three regions: The external ear, middle ear, and inner ear. A portion of the external ear along with the entirety of the middle and inner ear are located within the temporal bone of the skull.
Structures of the Ear
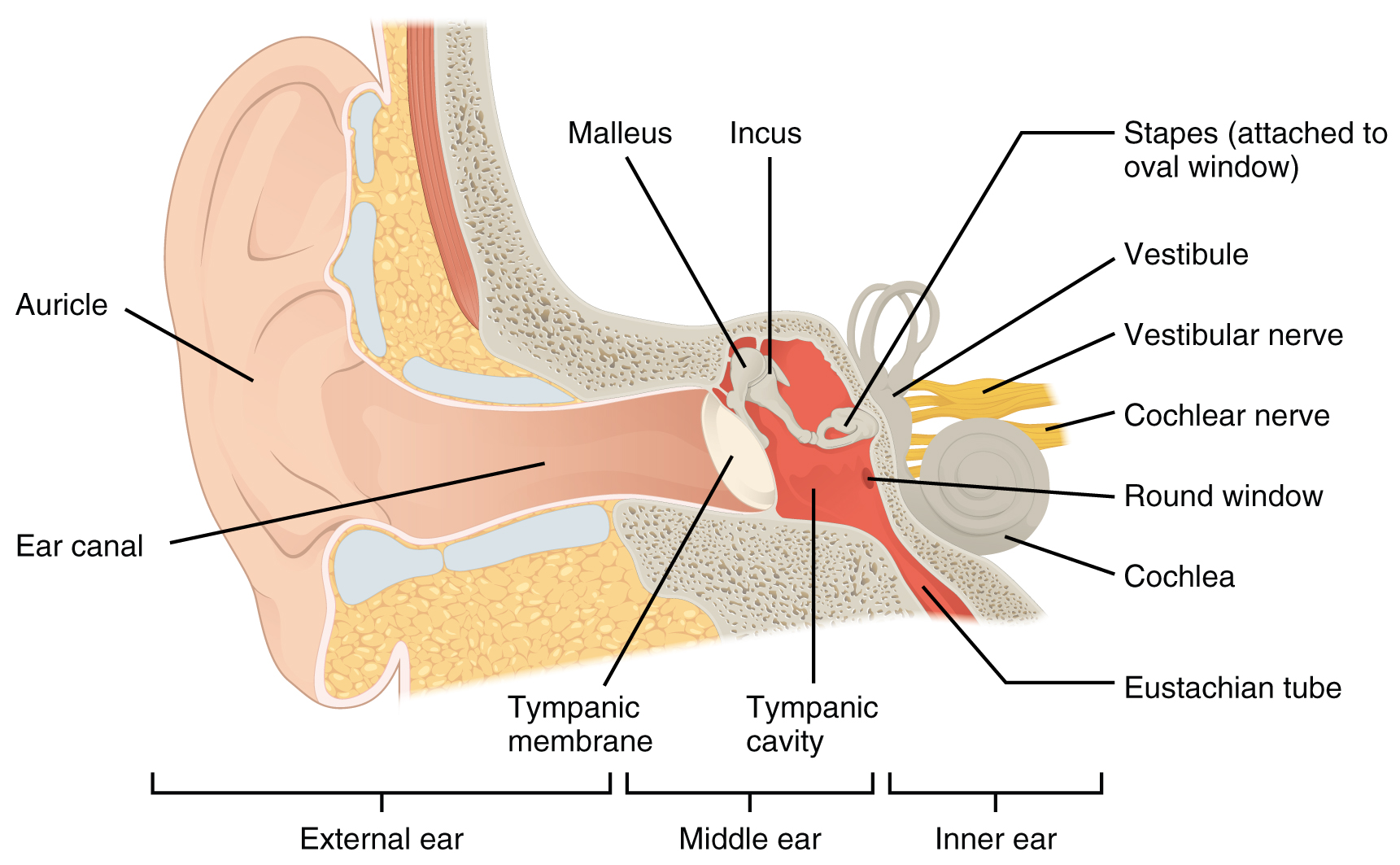
External (Outer) Ear
The external ear includes the auricle, external auditory meatus, and tympanic membrane. The external ear functions to collect sound and direct it to deeper structures of the ear and is only involved in the sensation of hearing.
The large, fleshy structure on the lateral aspect of the head is known as the auricle. This is also called the pinna. It is formed of elastic cartilage and functions to gather and amplify sound. The way the sound bounces off of the ridges of the auricle provides the brain with clues about the direction the sound came from.
The C-shaped curves of the auricle direct sound waves toward the external acoustic meatus (auditory canal). The canal enters the skull through the external auditory meatus of the temporal bone. Laterally it is formed by cartilage, while the medial two thirds is formed by the temporal bone.
At the end of the external acoustic meatus is a thin layer of tissue called the tympanic membrane, or eardrum, which vibrates in response to sound waves. The tympanic membrane separates the external ear from the middle ear.
Middle Ear
The middle ear contains the air-filled tympanic cavity and is located just medial to the tympanic membrane. This region is only involved in the sensation of hearing. It is spanned by three small bones called the auditory ossicles. The three ossicles are the malleus, incus, and stapes, which are Latin names that roughly translate to hammer, anvil, and stirrup. The malleus is attached to the tympanic membrane and articulates with the incus. The incus, in turn, articulates with the stapes. The stapes is then attached to the membrane covering the oval window, the superior opening between the middle and inner ear. When that membrane moves, sound information is transmitted to the inner ear.
The middle ear is connected to the pharynx through the auditory (Eustachian) tube, which helps equilibrate air pressure across the tympanic membrane. The tube is normally closed but will pop open when the muscles of the pharynx contract during swallowing or yawning.
Inner Ear
The inner ear has two sensory functions served by two structures. The cochlea is involved in the process of hearing and is attached to the stapes via the oval window. The vestibular complex is involved in the process of vestibulation and consists of the semicircular ducts involved in sensing rotational acceleration and the vestibule, consisting of the utricle and saccule, involved in sensing linear acceleration.
The structures of the inner ear can be divided into two divisions, the bony labyrinth, and the membranous labyrinth. The bony labyrinth is composed of a series of canals embedded within the temporal bone. The bony labyrinth contains perilymph and the inner membranous labyrinth. The membranous labyrinth contains endolymph and is where the gelatinous layers and specialized hair cells will be found that produce neural signals. The neural signals from these two regions are relayed to the brain stem through separate fiber bundles – vestibular and cochlear divisions. However, these two distinct bundles travel together from the inner ear to the brain stem as the vestibulocochlear nerve.
The round window is the inferior opening connecting the middle and inner ear. It is covered by a membrane that contains perilymph within the bony labyrinth. The membrane moves as sound waves within the perilymph reach it, allowing remaining energy to be dissipated and preventing echoes within the cochlea.
Audition (Hearing)
This content will be covered in lecture.
Hearing, or audition, is the transduction of sound waves into a neural signal. Sound waves enter the ear and travel through the external acoustic meatus. The sound waves move the tympanic membrane, and that movement results in the vibration of the auditory ossicles due to the connection of the malleus to the tympanic membrane. The stapes is connected to the membrane covering the oval window connecting the middle and inner ear. The oval window is located at the beginning of a fluid-filled (perilymph) tube within the cochlea called the scala vestibuli. The scala vestibuli extends from the oval window, traveling above the cochlear duct, which is the central cavity of the cochlea that contains the sound-transducing neurons. At the uppermost tip of the cochlea, the scala vestibuli curves over the top of the cochlear duct. The fluid-filled (perilymph) tube, now called the scala tympani, returns to the base of the cochlea, this time traveling under the cochlear duct. The scala tympani ends at the round window. As vibrations of the ossicles travel through the oval window, the perilymph of the scala vestibuli and scala tympani moves in a wave-like motion. The frequency of the fluid waves matches the frequencies of the sound waves. The membrane covering the round window will bulge out or pucker in with the movement of the perilymph within the scala tympani.
Transmission of Sound Waves to Cochlea
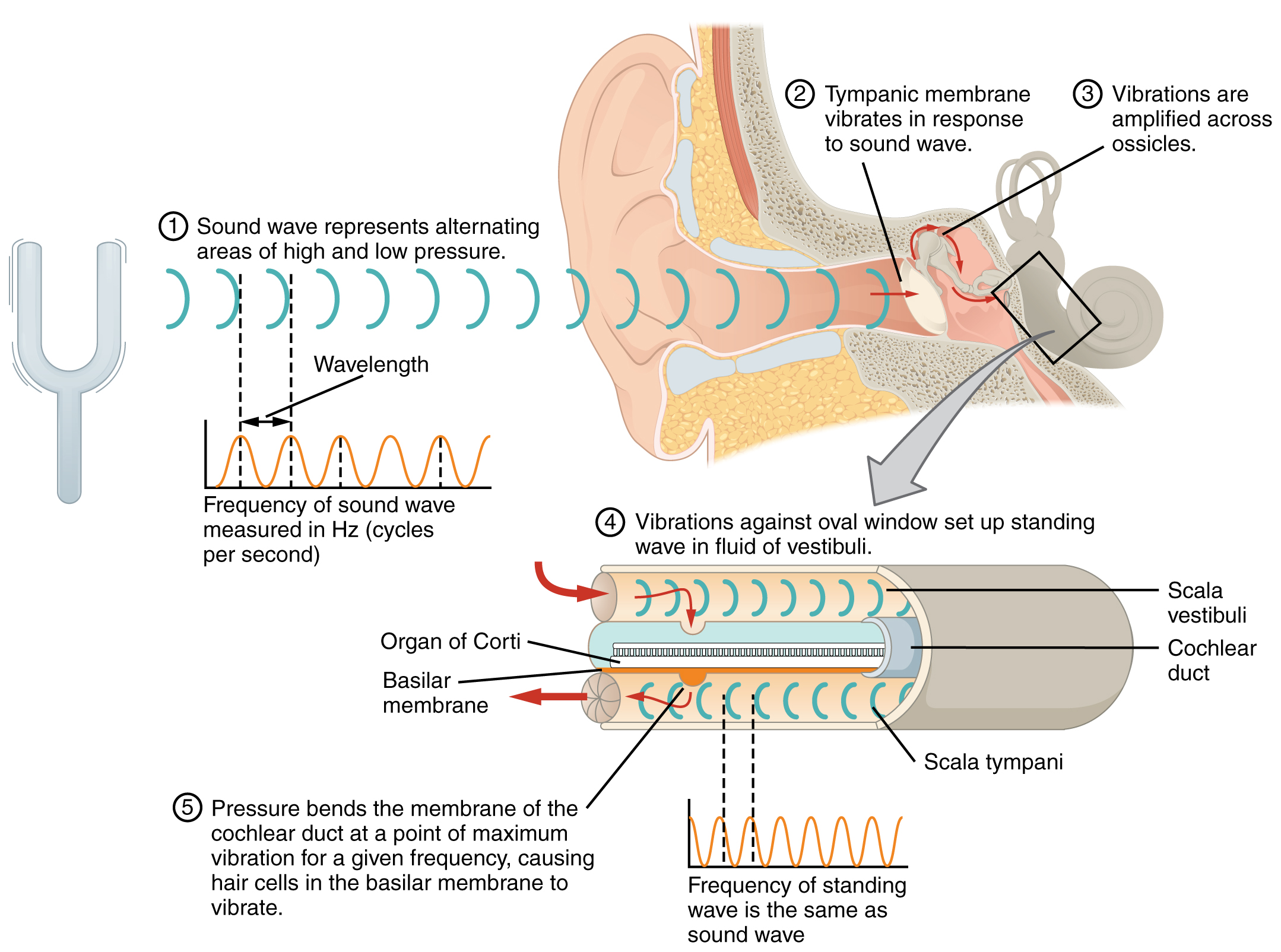
A cross-sectional view of the cochlea shows that the scala vestibuli and scala tympani run along both sides of the cochlear duct. The cochlear duct contains endolymph and the spiral organs (of Corti), which transduce the wave motion of the two scala into neural signals. The spiral organs lie on top of the basilar membrane, which is the side of the cochlear duct located between the spiral organs and the scala tympani. As the perilymph waves move through the scala vestibuli and scala tympani, the basilar membrane moves at a specific spot, depending on the frequency of the waves. Higher frequency waves move the region of the basilar membrane that is close to the base of the cochlea. Lower frequency waves move the region of the basilar membrane that is near the tip of the cochlea.
Cross Section of the Cochlea
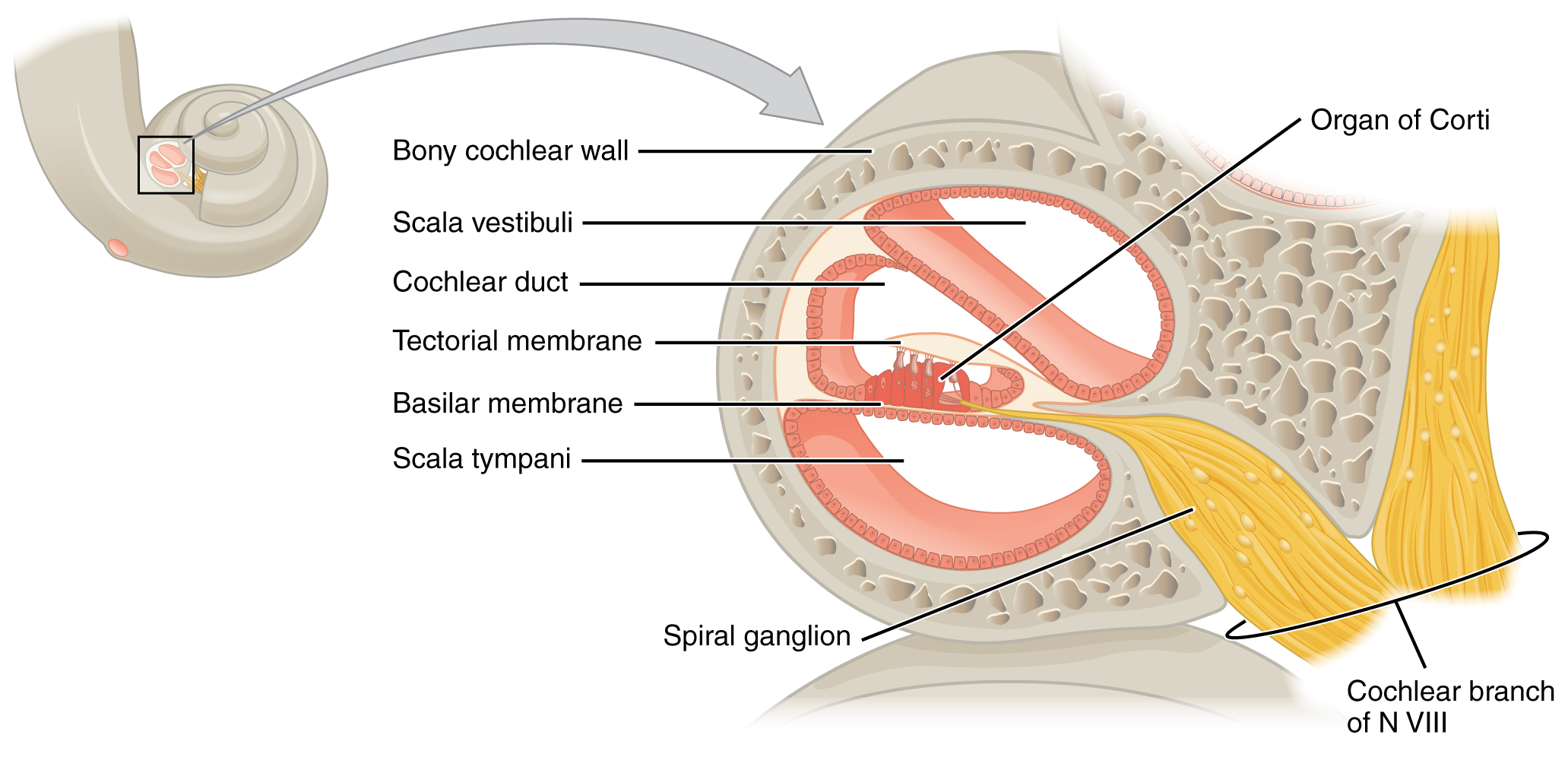
The spiral organs contain hair cells, which are named for the hair-like stereocilia extending from the cell’s apical surfaces. The stereocilia are an array of microvilli-like structures arranged from tallest to shortest. Protein fibers tether adjacent hairs together within each array, such that the array will bend in response to movements of the basilar membrane. The stereocilia extend up from the hair cells to the overlying tectorial membrane, which is attached medially to the spiral organ. When the pressure waves from the scala move the basilar membrane, the tectorial membrane slides across the stereocilia. The movement of the basilar membrane bends the stereocilia, depolarizing the hair cell membrane, triggering nerve impulses that travel down the afferent nerve fibers attached to the hair cells.
Hair Cell
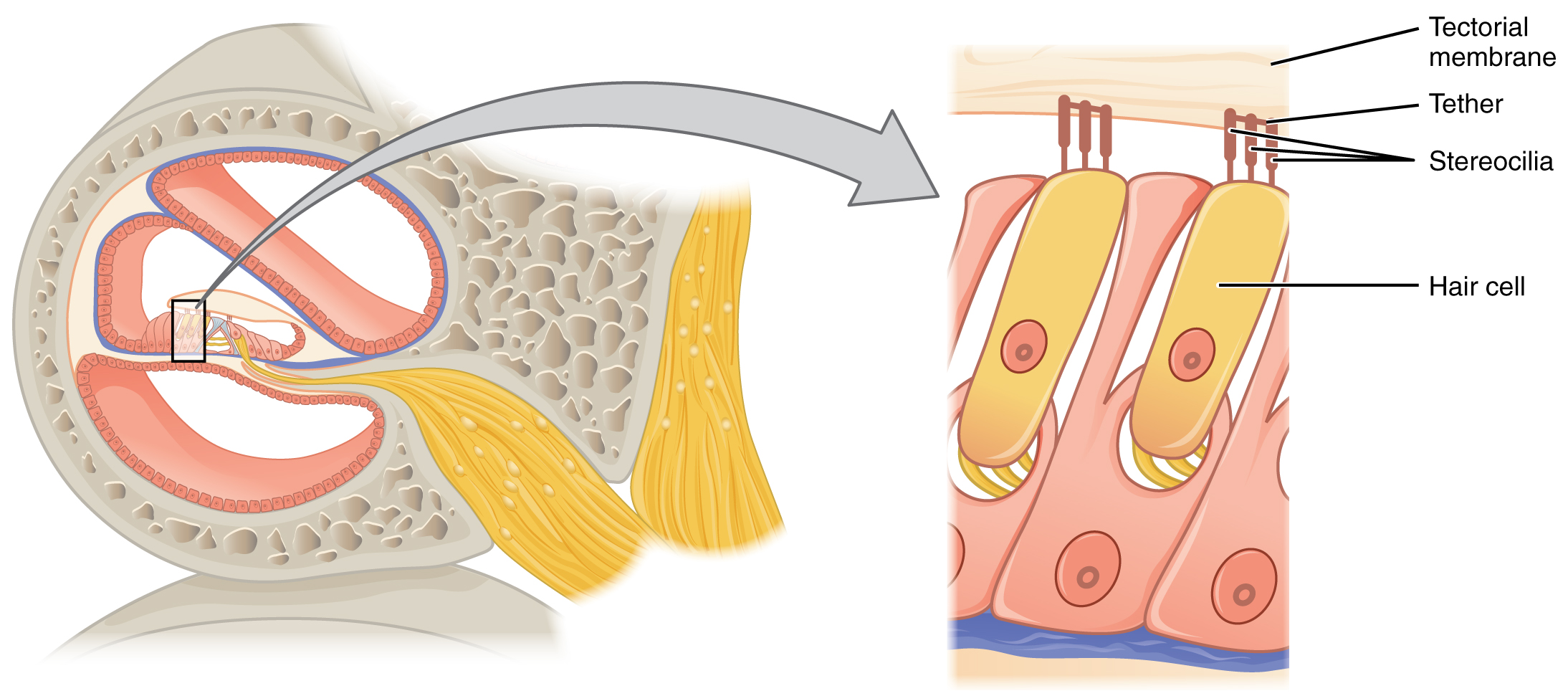
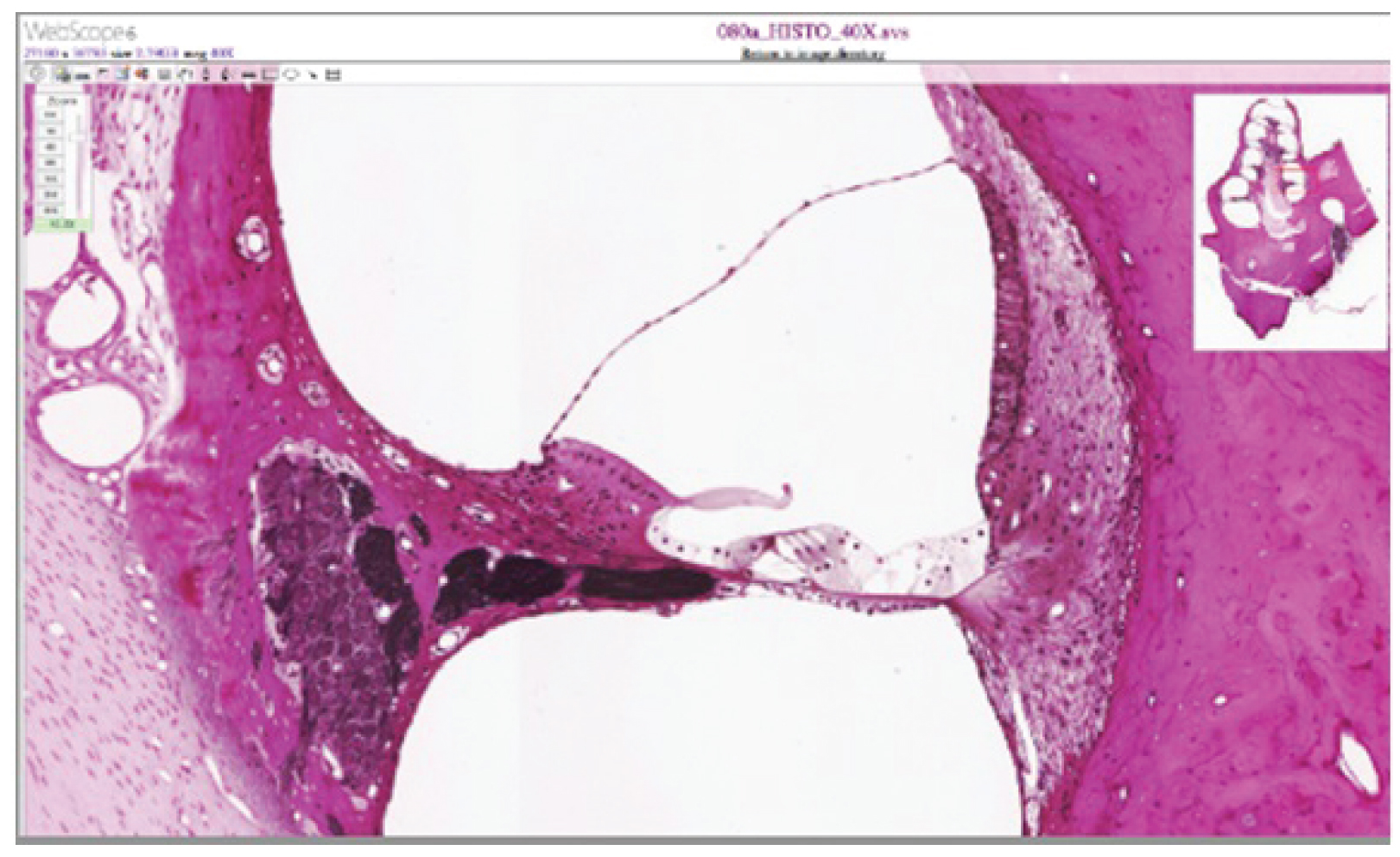
As stated above, a given region of the basilar membrane will only move if the incoming sound is at a specific frequency. Because the tectorial membrane only moves where the basilar membrane moves, the hair cells in this region will also only respond to sounds of this specific frequency. Therefore, as the frequency of a sound changes, different hair cells are activated all along the basilar membrane. The cochlea encodes auditory stimuli for frequencies between 20 and 20,000 Hz, which is the range of sound that human ears can detect. The unit of Hertz measures the frequency of sound waves in terms of cycles produced per second. Frequencies as low as 20 Hz are detected by hair cells at the apex, or tip, of the cochlea. Frequencies in the higher ranges of 20,000 Hz are encoded by hair cells at the base of the cochlea, close to the round and oval windows. Most auditory stimuli contain a mixture of sounds at a variety of frequencies and intensities (represented by the amplitude of the sound wave). The hair cells along the length of the cochlear duct, which are each sensitive to a particular frequency, allow the cochlea to separate auditory stimuli by frequency, just as a prism separates visible light into its component colors.
Frequency Coding in the Cochlea
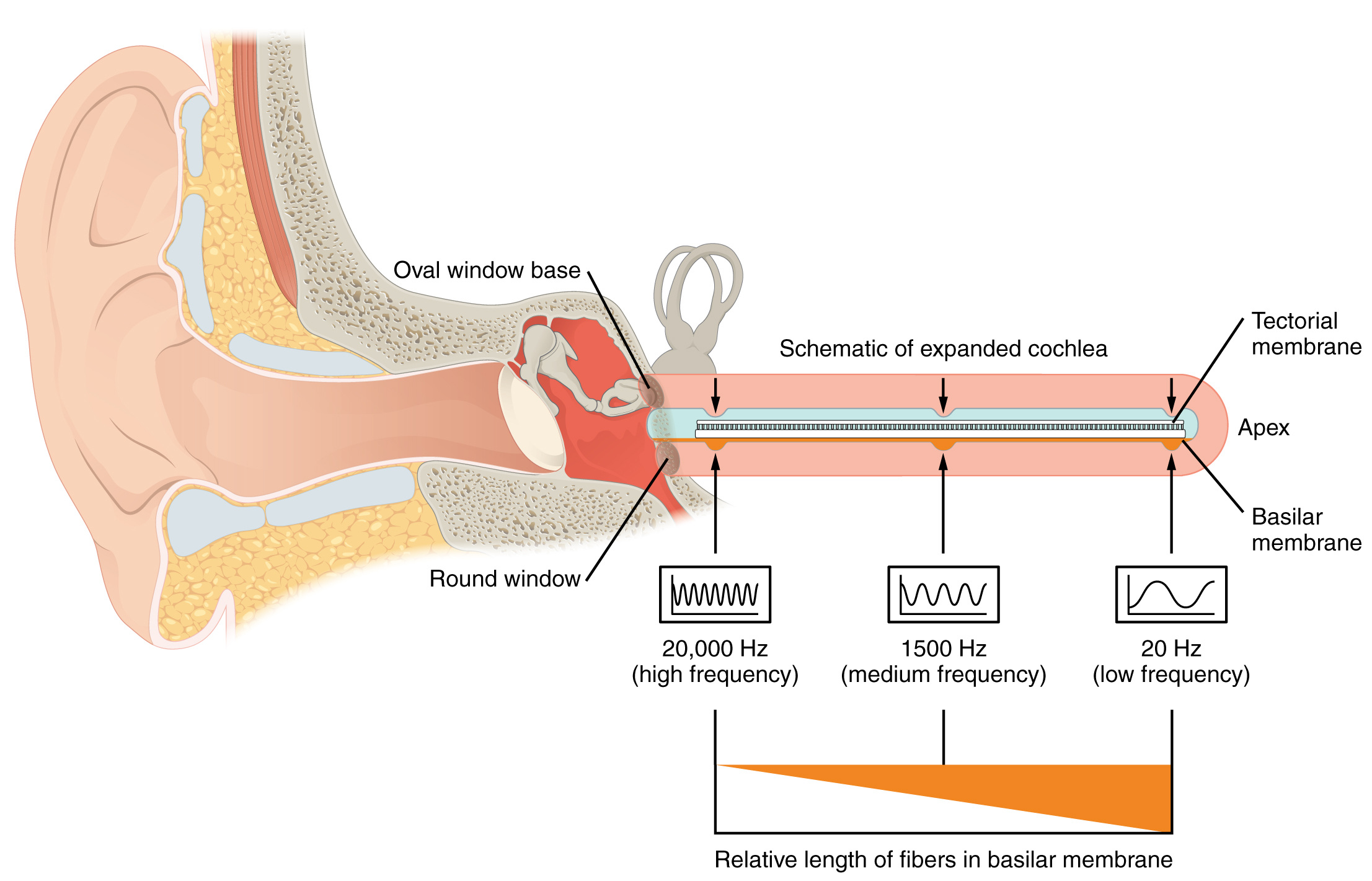
Vestibulation (Balance)
This content will be covered in lecture.
Along with audition, the inner ear is responsible for encoding information about vestibulation (equilibrium), the sense of balance, or spatial awareness. A similar mechanoreceptor—a hair cell with stereocilia—senses head position, head movement, and whether our bodies are in motion. These cells are located within the vestibule of the inner ear. Head position is sensed by the utricle and saccule, whereas head movement is sensed by the semicircular canals. The neural signals generated in the vestibular ganglion are transmitted through the vestibulocochlear nerve to the brain stem and cerebellum.
The utricle and saccule are both largely composed of macula tissue (plural = maculae). The macula is composed of hair cells surrounded by support cells. The stereocilia of the hair cells extend into a viscous gel called the otolithic membrane. On top of the otolithic membrane is a layer of calcium carbonate crystals, called otoliths. The otoliths essentially make the otolithic membrane top-heavy. The otolithic membrane moves separately from the macula in response to head movements. Tilting the head causes the otolithic membrane to slide over the macula in the direction of gravity. The moving otolithic membrane, in turn, bends the stereocilia, causing some hair cells to depolarize as others hyperpolarize. The exact position of the head is interpreted by the brain based on the pattern of hair-cell depolarization.
Linear Acceleration Coding by Maculae
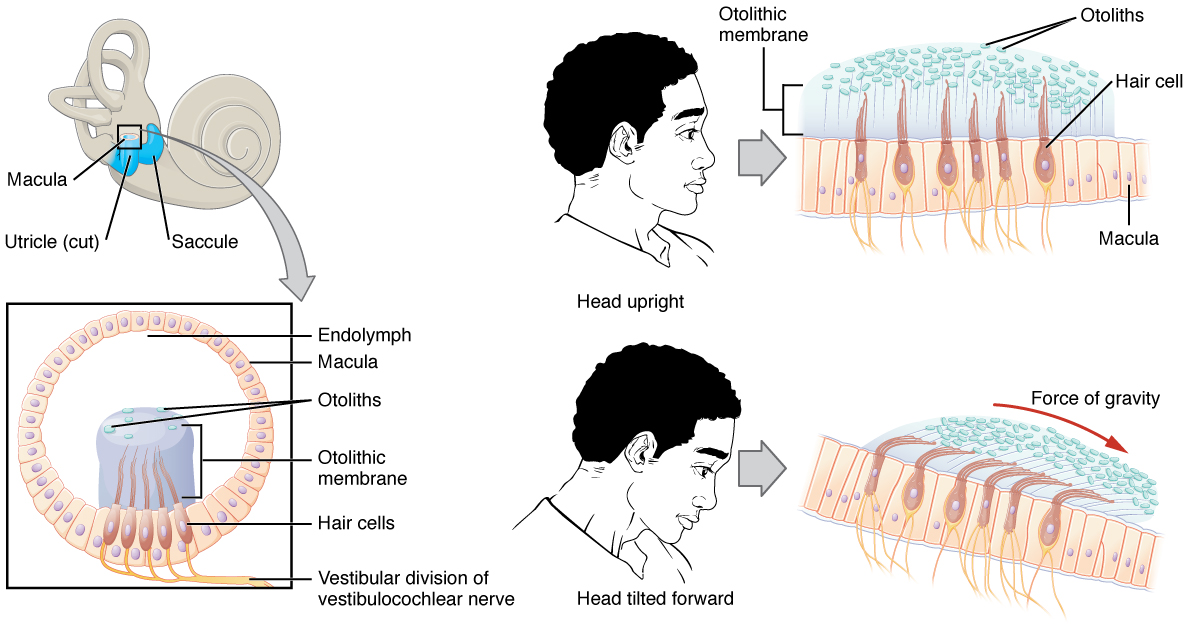
The semicircular canals are three ring-like extensions of the vestibule. One is oriented in the horizontal plane, whereas the other two are oriented in the sagittal and coronal planes. The base of each semicircular canal, where it meets with the vestibule, connects to an enlarged region known as the ampulla. The ampulla contains the hair cells that respond to rotational movements, such as turning the head while saying “no.” The stereocilia of these hair cells extend into the cupula, a membrane that attaches to the top of the ampulla. As the head rotates in a plane parallel to the semicircular canal, the fluid lags, deflecting the cupula in the direction opposite to the head movement. The semicircular canals contain several ampullae, with some oriented horizontally and others oriented vertically. By comparing the relative movements of both the horizontal and vertical ampullae, the vestibular system can detect the direction of most head movements within three-dimensional (3-D) space.
Rotational Coding by Semicircular Canals
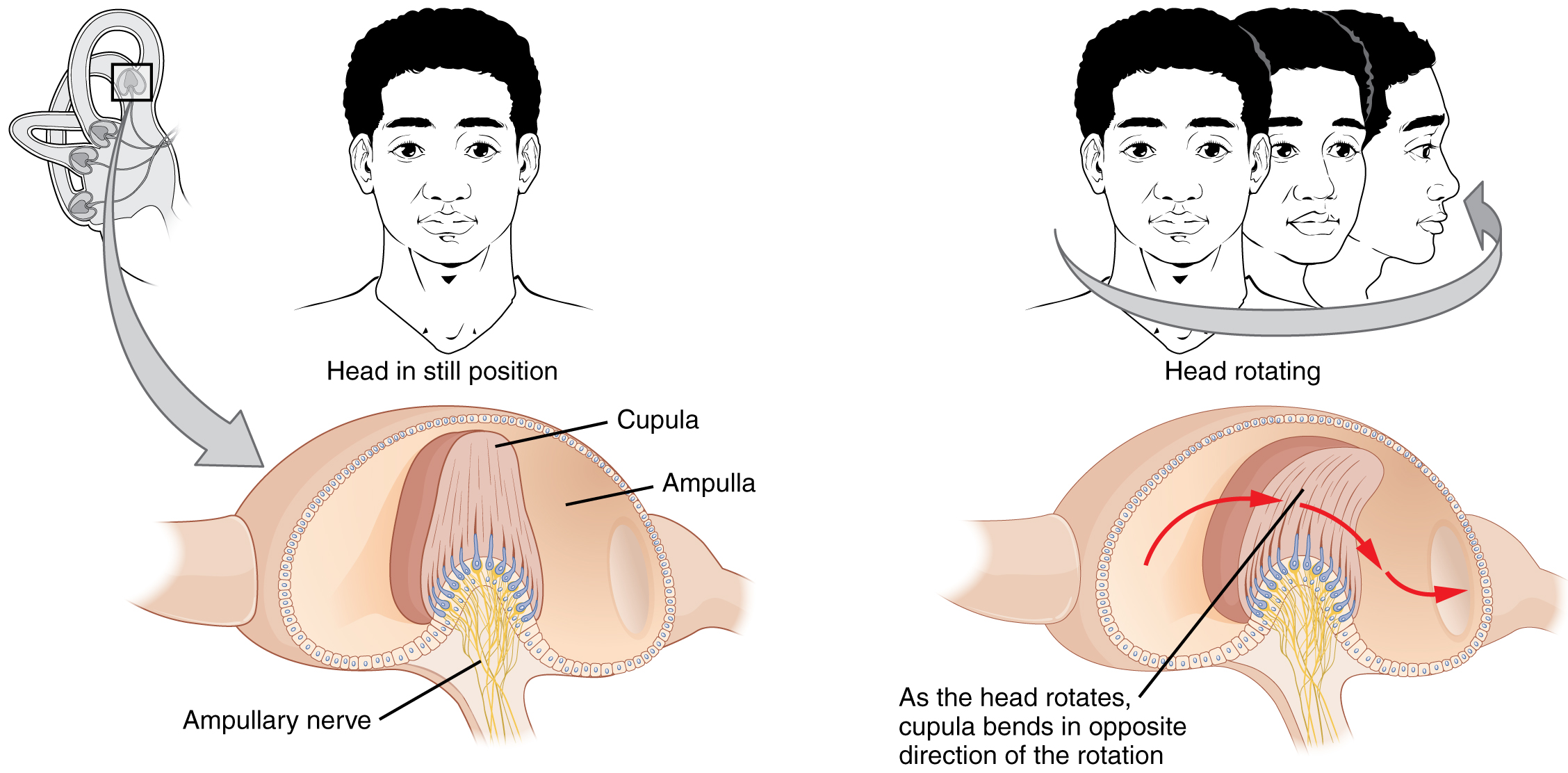
Auditory Pathways
The sensory pathway for audition travels along the vestibulocochlear nerve, which synapses with neurons in the cochlear nuclei of the superior medulla. Within the brain stem, input from either ear is combined to extract location information from the auditory stimuli. Whereas the initial auditory stimuli received at the cochlea strictly represent the frequency—or pitch—of the stimuli, the locations of sounds can be determined by comparing information arriving at both ears.
Sound Localization FYI – For Your Information (-> you will not be assessed on this content)
Sound localization is a feature of central processing in the auditory nuclei of the brain stem. Sound localization is achieved by the brain calculating the interaural time difference and the interaural intensity difference. A sound originating from a specific location will arrive at each ear at different times unless the sound is directly in front of the listener. If the sound source is slightly to the left of the listener, the sound will arrive at the left ear microseconds before it arrives at the right ear. This time difference is an example of an interaural time difference. Also, the sound will be slightly louder in the left ear than in the right ear because some of the sound waves reaching the opposite ear are blocked by the head.
Auditory Brain Stem Mechanisms of Sound Localization
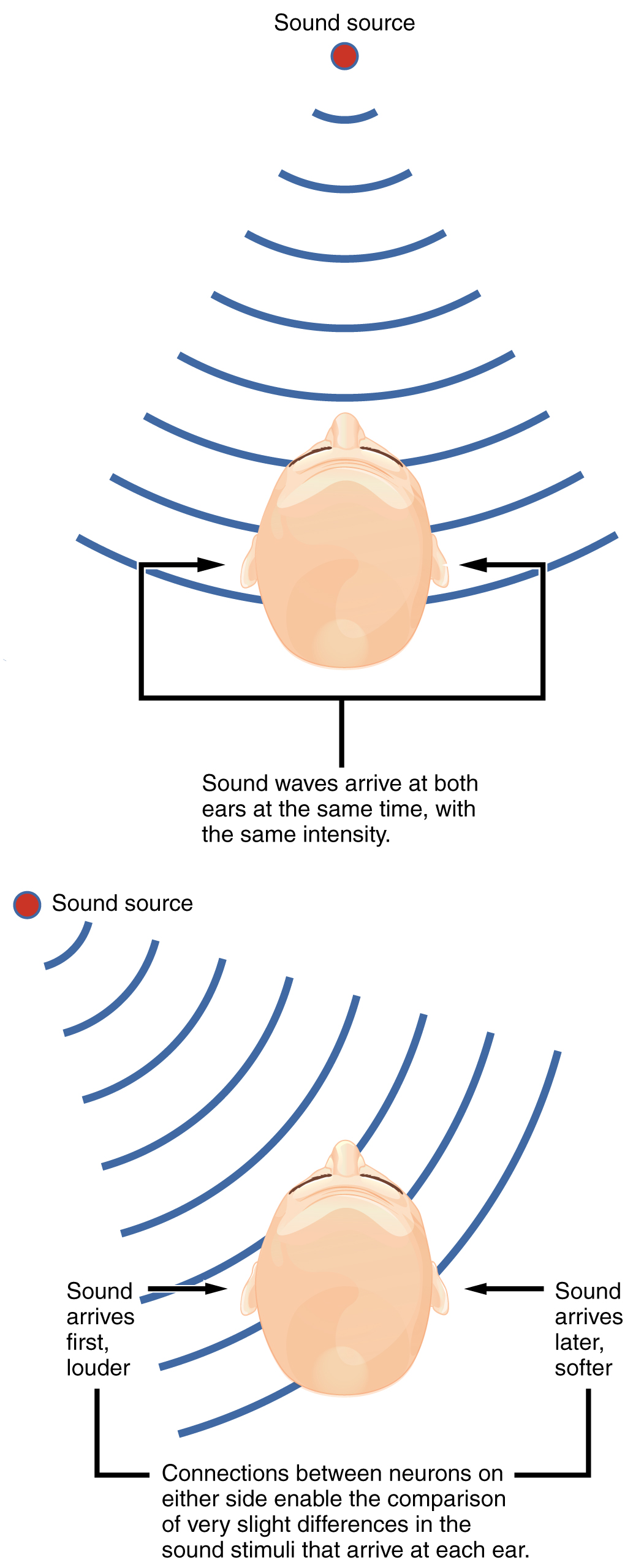
Auditory processing continues on to a nucleus in the midbrain called the inferior colliculus. Axons from the inferior colliculus project to two locations, the thalamus and the superior colliculus. The medial geniculate nucleus of the thalamus receives the auditory information and then projects that information to the auditory cortex in the temporal lobe of the cerebral cortex. The superior colliculus receives input from the visual and somatosensory systems, as well as the ears, to initiate stimulation of the muscles that turn the head and neck toward the auditory stimulus.
Vestibular Pathways
Balance is coordinated through the vestibular system, the nerves of which are composed of axons from the vestibular ganglion that carries information from the utricle, saccule, and semicircular canals. The system contributes to controlling head and neck movements in response to vestibular signals. An important function of the vestibular system is coordinating eye and head movements to maintain visual attention. Most of the axons terminate in the vestibular nuclei of the medulla. Some axons project from the vestibular ganglion directly to the cerebellum, with no intervening synapse in the vestibular nuclei. The cerebellum is primarily responsible for initiating movements on the basis of equilibrium information.
Neurons in the vestibular nuclei project their axons to targets in the brain stem. One target is the reticular formation, which influences respiratory and cardiovascular functions in relation to body movements. A second target of the axons of neurons in the vestibular nuclei is the spinal cord, which initiates the spinal reflexes involved with posture and balance. To assist the visual system, fibers of the vestibular nuclei project to the oculomotor, trochlear, and abducens nuclei to influence signals sent along the cranial nerves. Finally, the vestibular nuclei project to the thalamus to join the proprioceptive pathway of the dorsal column system, allowing conscious perception of equilibrium.
Vestibulo-ocular Reflex FYI – For Your Information (-> you will not be assessed on this content)
The connections of the vestibular nuclei to the extraocular muscles constitute the pathway of the vestibulo-ocular reflex (VOR), which compensates for head and body movement by stabilizing images on the retina.
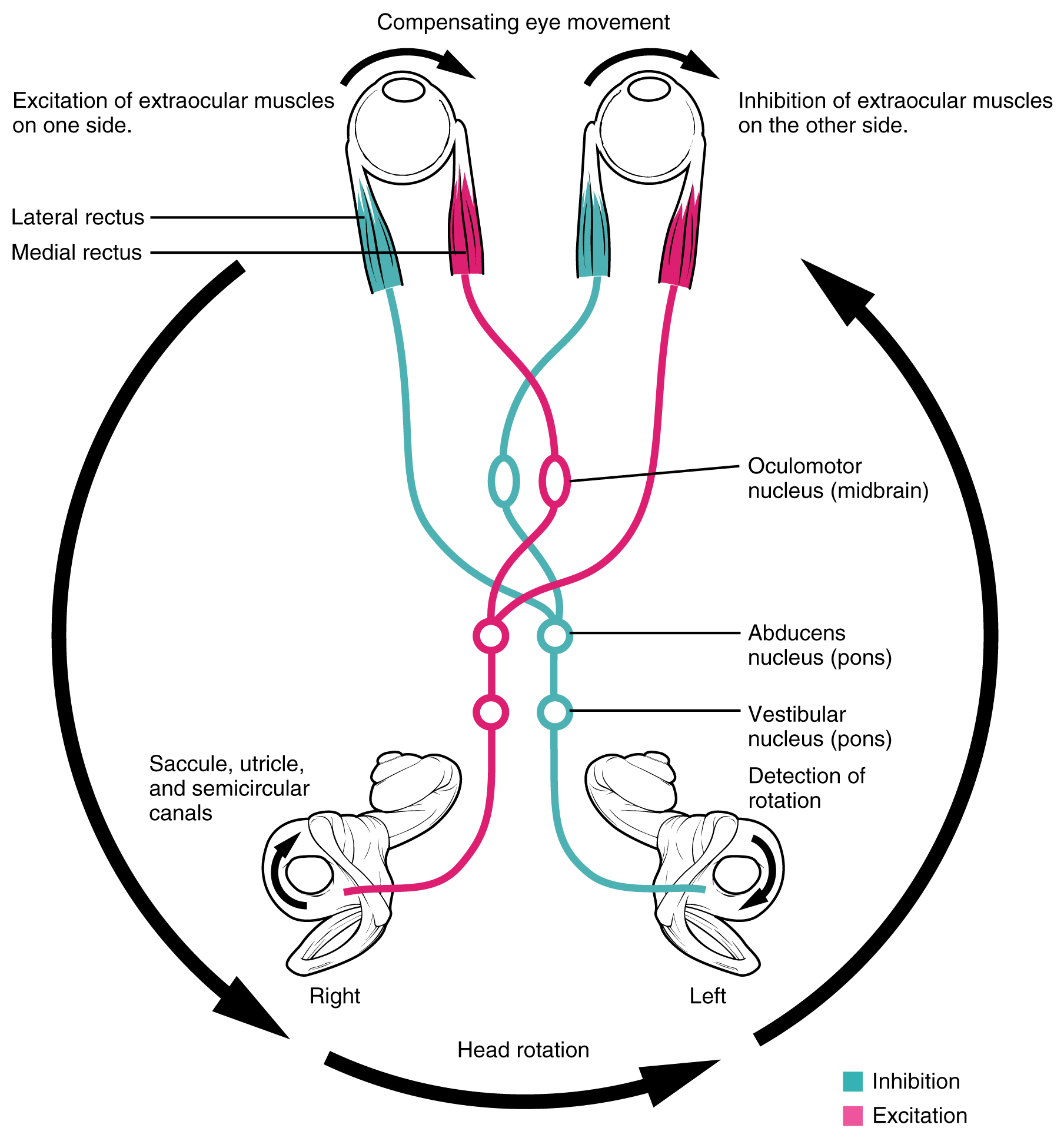