Unit Two
Day 14: Petrochemistry, Alcohols
As you work through this section, if you find that you need a bit more background material to help you understand the topics at hand, you can consult “Chemistry: The Molecular Science” (5th ed. Moore and Stanitski) Chapter 10-1 through 10-4, and/or Chapter 7.5-7.6 in the Additional Reading Materials section.
D14.1 Petroleum Chemistry
Petroleum consists primarily of naturally occurring hydrocarbons, predominantly alkanes and cycloalkanes. Molecules of these substances have negligible dipole moments and are essentially nonpolar. Hence, the dominant IMF present is London dispersion forces. The alkane chains can be quite long, and properties such as melting point and boiling point (Table 1) usually vary smoothly and predictably as a function of the number of carbon atoms in a series of linear alkane molecules.
Alkane* | Molecular Formula | Melting Point (°C) | Boiling Point (°C) | Phase at STP** | Number of Constitutional Isomers |
---|---|---|---|---|---|
methane | CH4 | –182.5 | –161.5 | gas | 1 |
ethane | C2H6 | –183.3 | –88.6 | gas | 1 |
propane | C3H8 | –187.7 | –42.1 | gas | 1 |
butane | C4H10 | –138.3 | –0.5 | gas | 2 |
pentane | C5H12 | –129.7 | 36.1 | liquid | 3 |
hexane | C6H14 | –95.3 | 68.7 | liquid | 5 |
heptane | C7H16 | –90.6 | 98.4 | liquid | 9 |
octane | C8H18 | –56.8 | 125.7 | liquid | 18 |
nonane | C9H20 | –53.6 | 150.8 | liquid | 35 |
decane | C10H22 | –29.7 | 174.0 | liquid | 75 |
tetradecane | C14H30 | 5.9 | 253.5 | solid | 1858 |
octadecane | C18H38 | 28.2 | 316.1 | solid | 60,523 |
Table 1. Properties of Some Alkanes
*Physical properties for C4H10 and heavier molecules are those of the normal isomer, n-butane, n-pentane, etc. **STP indicates a temperature of 0 °C and a pressure of 1 atm. |
As the number of carbon atoms increases, the number of constitutional isomers increases dramatically. The constitutional isomers have different physical properties: branched alkane isomers have different melting and boiling points from linear alkanes, for example.
The most important reaction of alkanes is combustion. The simplest example is combustion of methane:
CH4(g) + 2O2(g) → CO2(g) + 2H2O(g) ΔrH = −802.3 kJ/mol
Combustion of alkanes is highly exothermic so alkanes are excellent fuels. For example, methane is the principal component of natural gas and butane is used in camping stoves and lighters. Gasoline is a liquid mixture of linear and branched alkanes, each containing five to twelve carbon atoms, plus various additives to improve its performance as a fuel. Kerosene, diesel fuel, motor oil, and fuel oil are primarily mixtures of alkanes with higher molecular masses than gasoline. Provided there is plenty of oxygen available, almost all of the carbon in the alkane fuel is converted to carbon dioxide. Thus combustion of alkanes invariably adds water vapor and CO2 to the atmosphere—a human contribution to global warming.
The main source of alkane fuels is petroleum (from Latin, petra: “rock”, oleum: “oil”) or crude oil, a complex mixture that can be separated by fractional distillation. Fractional distillation takes advantage of the boiling point differences of the various component substances (Figure 1), which arises from the differences in their intermolecular forces.
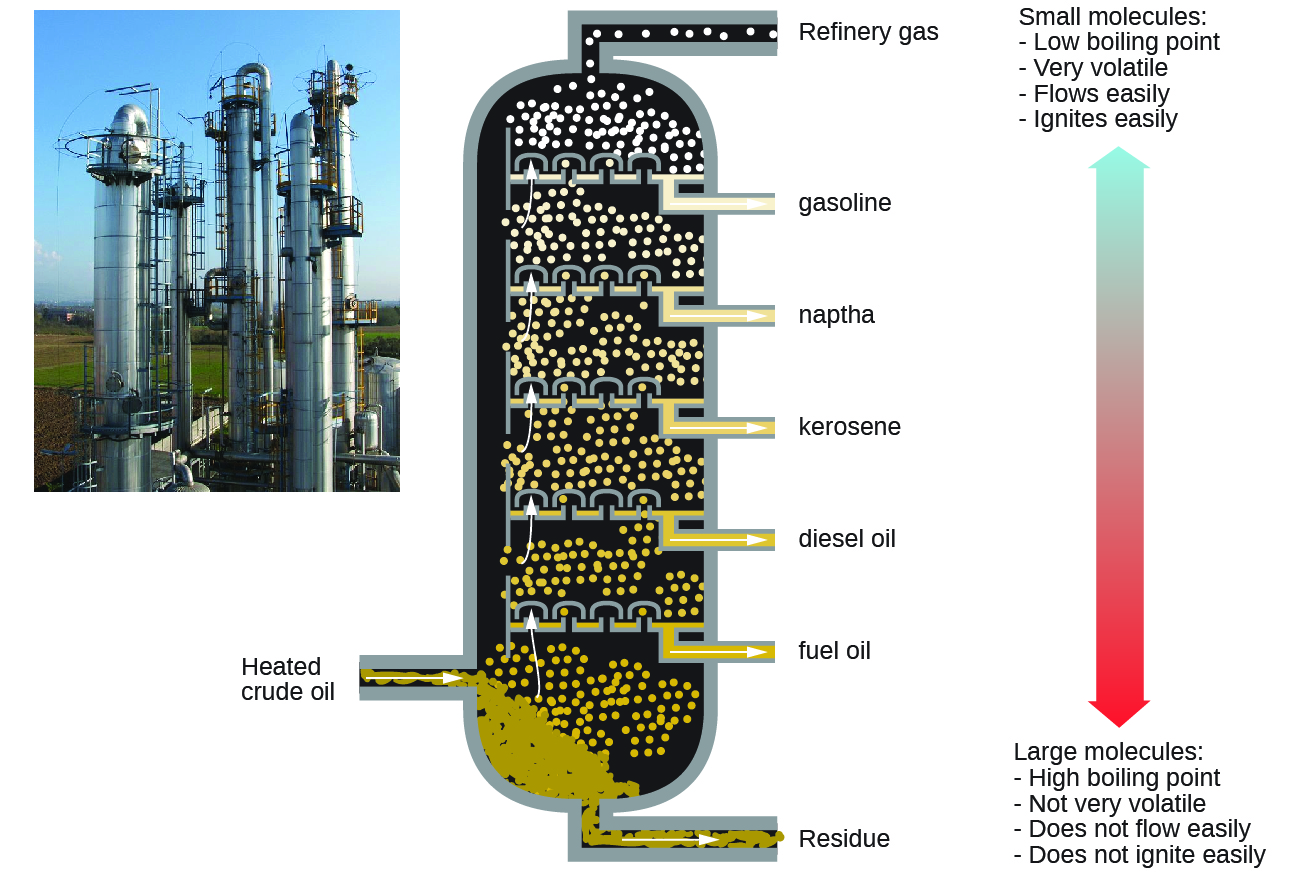
Because there is greater demand for gasoline than for other components of petroleum, catalytic cracking is used in petroleum refining to break up larger molecules into smaller molecules, some of which are within the gasoline range of 5–12 carbon atoms. Cracking can occur at high pressures and temperatures without a catalyst or at less harsh conditions by using a catalyst. The hydrocarbon molecules are broken up in a fairly random way to produce mixtures of smaller molecules, some of which have carbon-carbon double bonds. One possible reaction involving C15H32 might be:
C15H32 → 2 C2H4 (ethene) + C3H6 (propene) + C8H18 (octane)
The alkene products, ethene and propene, are important for producing other organic chemicals. The octane product is a component of gasoline.
D14.2 Functional Groups
With over twenty million known organic compounds in existence, it would be very challenging to memorize chemical reactions for each one. Fortunately, molecules with similar functional groups tend to undergo similar reactions. A functional group is defined as an atom or group of atoms within a molecule that has similar chemical properties whenever it is present in a molecule Even if other parts of a molecule are quite different, a specific functional group usually reacts the same way.
You may want to review the discussion of alkanes in Sections 7.4 and 7.5. Because C–C and C–H bonds are strong, alkanes are unreactive at room temperature. High temperatures or high-energy photons can cause alkanes to react, so we primarily use alkanes as fuels. An alkyl group is a portion of an alkane molecule bonded to something else. Examples of alkyl groups are CH3–, methyl (part of a methane molecule with a bond), CH3CH2–, ethyl (derived from ethane), propyl, CH3CH2CH2–, and 2-propyl, (CH3)2CH–). (Both propyl and 2-propyl are derived from propane, with the bond in a different position.) If we want to designate any alkyl group, such as methyl, or ethyl, or any other, we use R (for the Rest of the molecule). When there are two or more different alkyl groups we use R, R’, R”, etc. For example, R and R’, are trans to each other in the structure below:
Alkenes (Sections 8.3 and 8.4) have the C=C functional group and alkynes (Section 8.5) have a carbon-carbon triple bond as a functional group. Compounds in both of these classes are more reactive than alkanes, because the double and triple bonds can be partly broken (a π bond can break) more easily than a C–C or C–H bond can be broken. Both alkenes and alkynes can undergo addition reactions, for example, where the sigma bond remains intact, but the electrons in the pi bond pair with two other atoms.
Next we will consider function groups that contain heteroatoms: atoms other than carbon and hydrogen, such as N, O, or S. These functional groups impart specific reactivity to a molecule and also affect IMFs present, giving rise to differing physical properties.
D14.3 Alcohols
All alcohol molecules have one or more –OH (hydroxyl) functional groups covalently bonded to a carbon atom. (Note that the –OH group is covalently bonded—it is not a hydroxide ion.) The smallest alcohol is methanol (CH3OH). Ethanol is an alcohol produced by some species of yeast via fermentation of various sugars:
Ethanol can also be synthesized using the addition reaction of ethene and water using an acid as a catalyst:
Exercise 1: Structures of Alcohols
While you will not be explicitly tested on nomenclature, it is still useful to know how to name molecules. See the nomenclature appendix for additional descriptions on how to name alcohol compounds.
D14.4 Intermolecular Forces in Alcohols
In the alcohol functional group, the C–O and O–H bonds are at approximately a tetrahedral angle and O–H……:O hydrogen bonds can form, so the-OH functional group makes alcohol molecules polar and capable of hydrogen bonding. Hence, the IMFs present in alcohol are London dispersion forces, dipole-dipole attractions, and hydrogen bonding. For example, 1-butanol (CH3CH2CH2CH2OH) and pentane (CH3CH2CH2CH2CH3) have the same number of electrons and a similar molecular shape. But the boiling point of 1-butanol is 117 °C, considerably higher than that of pentane, 36.3 °C. This difference can be attributed to hydrogen bonding, dipole-dipole attractions, in addition to London forces in 1-butanol compared to London forces only in pentane.
London dispersion forces depend on compactness of a molecule and that can affect properties of isomeric alcohols. For example, even though they are isomers, 2-methylpropan-1-ol, (CH3)2CHCH2OH, has a lower boiling point (108 °C) than 1-butanol, because branching makes the molecule more compact and lowers the strength of London dispersion forces.
The -OH end of an alcohol molecule is hydrophilic (“water-loving”), capable of strong intermolecular interactions with water molecules. The dipole-dipole and hydrogen-bond interactions between alcohol molecules and water molecules are similar in strength to those between alcohol molecules and alcohol molecules or between water molecules and water molecules. The hydrocarbon (alkyl) end of an alcohol molecule is essentially nonpolar and is hydrophobic (“water-fearing”), where the intermolecular interactions between hydrophobic molecules and water molecules are weak compared to water-water or hydrocarbon-hydrocarbon interactions.
Hence, although the size of the alkyl group has little influence the reactivity of an alcohol molecule, it has a significant impact on the solubility of alcohols in water. Alcohols with small alkyl groups, e.g. methanol and ethanol, are completely miscible with water, which means they have infinite mutual solubility. Alcohols with larger molecules, such as 1-octanol, are immiscible in water, which means that when 1-octanol is added to water two layers form. One layer is nearly pure water and the other layer nearly pure 1-octanol, because the solubility of each substance in the other is very low.
D14.5 Oxidation Reactions of Alcohols
Alcohols can be classified according to the number of alkyl groups attached to the carbon with the -OH group. If a hydrogen atom or one alkyl group is attached to that carbon, the alcohol is a primary (1º) alcohol. If two alkyl groups are attached, the alcohol is a secondary (2º) alcohol. If three alkyl groups are attached, the alcohol is a tertiary (3º) alcohol (Figure 2).
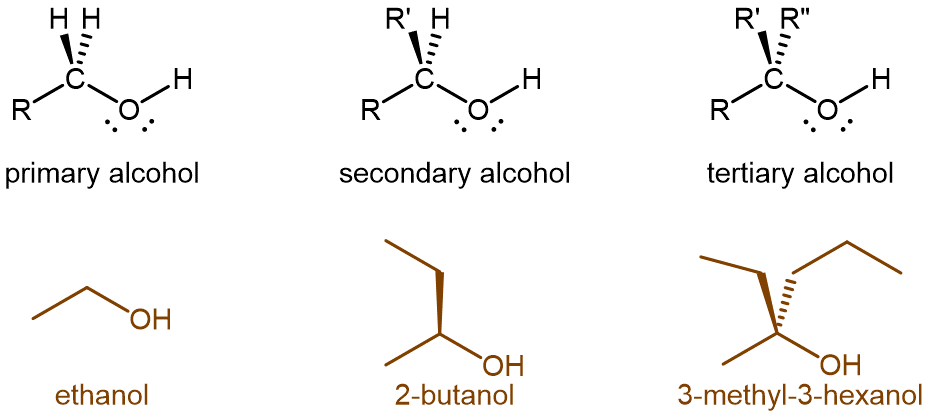
All alcohols are combustible, but controlled oxidation is even more important than combustion because it can convert the alcohol functional group into other functional groups. The ease with which an alcohol can be oxidized and the extent of the oxidation depends on whether the alcohol is primary, secondary, or tertiary.
Oxidation of an organic compound can usually be recognized because either an oxygen atom is added to the reactant molecule or two hydrogen atoms are lost from the reactant molecule. For a primary alcohol (e.g. ethanol), controlled oxidation first produces an aldehyde (e.g. ethanal or acetaldehyde); an aldehyde has the functional group –CHO. Then the aldehyde is further oxidized to a carboxylic acid (e.g. ethanoic acid or acetic acid); a carboxylic acid has the functional group –COOH.
Common oxidizing agents used in the laboratory for controlled oxidation are aqueous solution of potassium permanganate (KMnO4(aq)) or aqueous acidic solution of potassium dichromate (K2Cr2O7(aq)).
For a secondary alcohol (e.g. 2-propanol), the oxidation product is a ketone (e.g. propanone or acetone); ketones have a functional group that consists of a C=O group with two alkyl groups attached to the C atom.
Ketones are difficult to oxidize further because, without breaking C–C bonds, there is no obvious way to add one more oxygen atom to the carbon atom in the C=O group and there are no hydrogen atoms to remove from that carbon atom.
Tertiary alcohols, with no hydrogen atoms attached to the carbon atom that is bonded to the –OH group, are difficult to oxidize. Tertiary alcohols do undergo combustion (to yield CO2 and H2O as hydrocarbons, primary alcohols, and secondary alcohols do), but they usually do not undergo controlled oxidation.
Exercise 2: Identifying Alcohols from Structure
Podia Question
Consider the structures shown here. List all substances that fall into each of the five categories below and explain why each substance fits each category you placed it in. Also explain why the other substances do not fit each category.
1. Contains a secondary alcohol functional group.
2. Is less soluble in water than 1-hexanol.
3. Has a higher boiling point than 1-hexanol.
4. Reacts with K2Cr2O7(aq) to form a carboxylic acid.
5. Undergoes addition of water (with an acid catalyst) to form a secondary alcohol.
Two days before the next whole-class session, this Podia question will become live on Podia, where you can submit your answer.