Unit Two
Day 16: Addition and Condensation Polymers
As you work through this section, if you find that you need a bit more background material to help you understand the topics at hand, you can consult “Chemistry: The Molecular Science” (5th ed. Moore and Stanitski) Chapter 10-6a through 10-6b, and/or Chapter 8.2-8.3 in the Additional Reading Materials section.
D16.1 Polymer Structure and Properties
A polymer consists of a tangle of long-chain molecules. If the intermolecular forces between the chains are smaller, so that it is easier for the molecules to move past one another, the polymer will be softer and will soften (melt) at lower temperature. If the intermolecular forces between the chains are sufficiently strong to prevent motion of the molecules past one another the polymer will be harder and higher melting.
Table 1 lists monomers for some well-known addition polymers and also some of their uses. You can probably find at least one example of each of them in your home. Each monomer is a variation of the structure of ethene (ethylene) in which one or more H atoms has been replaced by another group. By varying the nature of the highlighted group(s) in the monomer, the physical properties of the polymer, such as density, melting point, and hydrophobicity, can be controlled rather precisely and the polymer tailored for specialized uses. Note that in the equation below the polymer structure is specified by enclosing the repeating unit in brackets and specifying the number of repeating units by a subscript n.
Monomer | Common Name | Polymer | Some Typical Uses |
---|---|---|---|
![]() |
Ethylene | Polyethylene | Film for packaging and bags, toys, bottles, coatings |
![]() |
Propylene | Polypropylene (Herculon) | Milk cartons, rope, outdoor carpeting |
![]() |
Styrene | Polystyrene (Styrofoam, Styron) | Transparent containers, plastic glasses, refrigerators, styrofoam |
![]() |
Vinyl chloride | Polyvinyl chloride (PVC) | Pipe and tubing, raincoats, curtains, phonograph records, luggage, floor tiles |
![]() |
Vinylidene chloride | Polyvinylidene chloride (Saran) | Clinging food wrap |
![]() |
Acrylonitrile | Polyacrylonitrile (Orlon, Acrilan) | Textiles, rugs |
![]() |
Tetrafluoroethylene | Polytetrafluoroethylene (Teflon) | Nonstick pan coatings, bearings, gaskets |
![]() |
Vinyl acetate | Polyvinyl acetate | Elmer’s glue, wood glue |
![]() |
Methyl methacrylate | Polymethyl methacrylate (Plexiglass, Lucite) | Stiff, clear, plastic sheets, blocks, tubing, and other shapes |
Polymer properties can also be affected by cross linking. A cross-link is a covalent σ bond between one polymer chain and another that is not at the end of either chain. Cross links increase the molecular weight and limit the motions of the chains with respect to one another. With enough cross links a sample of a polymer could become a three-dimensional network held by sigma bonds—a single gigantic molecule. Another way to think about cross links is that, because they are covalent bonds, they are stronger than intermolecular forces between polymer chains and therefore amplify the effect of increasing intermolecular forces on polymer properties.
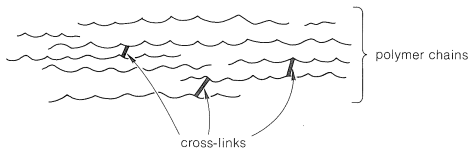
Exercise 1: Identifying a Monomer from a Polymer Structure
Consider this polymer:
Which monomer can produce this polymer?
D16.2 Conjugated Diene Polymers
Conjugated dienes (alkenes with two double bonds and a single bond in between) can be polymerized to form important substances, such as rubber, in nature and in the laboratory. The simplest conjugated diene is 1,3-butadiene. Figure 2 shows the 1,4-polymerization of this monomer, where a new σ covalent bond (highlighted in red) is formed between carbon 1 of one molecule and carbon 4 of another molecule. One electron from each of the π bonds pairs with an electron from an adjacent molecule to form two new σ bonds; the other electron from each π bond moves to the center of the molecule, between carbon 2 and carbon 3, forming a π bond at that position. In each equation, only two repeating units of the polymer are shown.
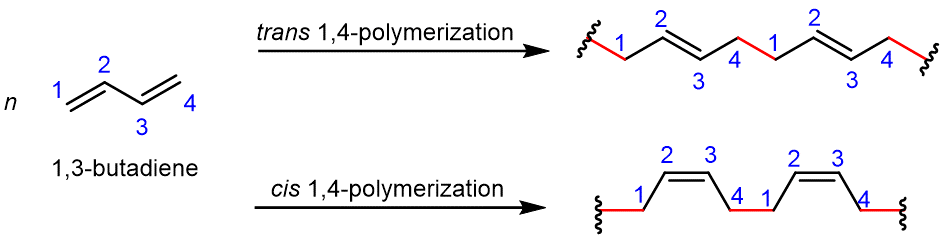
Natural rubber is formed from the monomer isoprene (2-methyl-1,3-butadiene), and is obtained as a milky white fluid known as latex. Most of the double bonds in the polymer chain have the cis configuration.
Chemical reactions involving double bonds on adjacent polymer chains leads to cross-linking, which enhances elasticity of the polymer. In 1839, Charles Goodyear discovered that when natural rubber was heated to 140–160 °C in the presence of sulfur, the rubber became tougher, more resistant to heat and cold, and more elastic This process was later called vulcanization after the Roman god of fire and volcanos, Vulcan. Above 140 °C, S–S bonds in sulfur molecules, S8, break and linear chains of sulfur atoms form. These chains then react with some of the remaining double bonds in the polymer, forming cross links. The development of vulcanized rubber for automobile tires greatly aided the automobile industry.
Another important conjugated diene used in synthetic rubber is chloroprene (2-chloro-1,3-butadiene). Polymerized chloroprene was developed by DuPont and given the trade name Neoprene. Cross-linking in polychloroprene involves combination of two chlorine atoms from adjacent chains with a Zn2+ ion to form ZnCl2. The C–Cl bonds in the uncross-linked polymer become C–C bonds—the cross-link. Cross-linking contributes to the overall elasticity of neoprene.
D16.3 Copolymers
Some of the most commercially important addition polymers are copolymers, made by polymerizing a mixture of two or more monomers. The properties of a copolymer are different from those of a mixture of the the polymers produced by the two or more monomers that went into the copolymer. Styrene-butadiene rubber (SBR), which is a copolymer of 1,3-butadiene and styrene mixed in about a 3:1 ratio, was developed in the U.S. during World War II when important supplies of natural rubber were cut off.
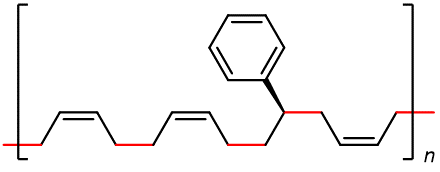
Styrene-butadiene rubber is more resistant to abrasion and oxidation than natural rubber and can also be vulcanized. More than 40% of the synthetic rubber production is SBR, which is used in tire production. Other copolymer examples include nitrile rubber, which is copolymerized from 1.3-butadiene and acrylonitrile (H2C=CHCN), and butyl rubber, which is copolymerized from isobutylene (H2C=C(CH3)2) and a small percentage of isoprene.
D16.4 Condensation Polymers: Polyesters and Polyamides
A condensation polymer is a polymer formed via a condensation reaction. The very small molecule produced in the condensation can be H2O, HCl, or some other simple molecule. Condensation polymerizations often, but not always, combine two different monomers in an alternating structure. Polymers usually grow by C-O or C-N bond formation; that is, an ester or amide linkage forms. The monomers typically have one functional group at each end; once one end of a monomer reacts, the second functional group remains and enables further lengthening of the polymer chain.
Polyesters
A polyester is a polymer where the individual units are held together by ester linkages. For example, the common polyester products Dacron, Terylene, and Mylar consist of polymer molecules formed from terephthalic acid (benzene-1,4-dicarboxylic acid) and ethylene glycol (ethane-1,2-diol) in a series of condensation reactions:

The usual name of this polyester is poly(ethylene terephthalate), PET. The repeating unit has the form
The presence of polar functional groups on the polymer chain often enhances chain-chain attractions, and thereby crystallinity and tensile strength. Polyester molecules make excellent fibers and are used in many fabrics. A knitted polyester tube, which is biologically inert, can be used in surgery to repair or replace diseased sections of blood vessels. PET is used to make bottles for soda pop and other beverages.
Polyamides
A polyamide is a polymer in which the individual units are held together by amide linkages. For example, nylon-66 is obtained from the reaction between 1,6-hexanediamine and adipic acid, each of which have 6 C atoms per molecule. (The first 6 is for the diamine and the second for the acid.) Other nylons have different numbers of C atoms in the monomer molecules, such as nylon-6 10, which has 6 C atoms in the diamine and 10 C atoms in the diacid. The monomers combine via condensation reactions to form amide linkages (highlighted below):
Nylon makes extremely strong threads and fibers because, in addition to London dispersion forces, there are dipole-dipole attractions and hydrogen bonding between the polymer chains (between a N-H in one strand and a lone pair in a C=O group in a neighboring strand). If you pull on both ends of a nylon thread, after a slight stretch, it will resist breaking because of the strong IMFs that hold neighboring chains together.
Kevlar (Figure 5) is a synthetic polymer made from 1,4-phenylene-diamine and terephthaloyl chloride. The material has a high tensile strength-to-weight ratio (it is about 5 times stronger than an equal weight of steel), making it useful for many applications from bicycle tires to sails to body armor.
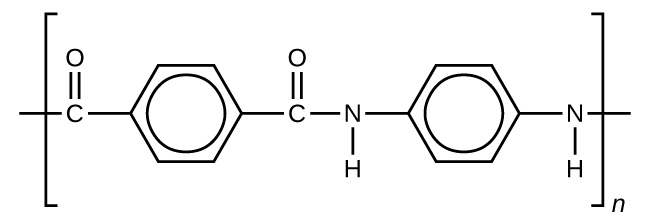
Similar to nylon, part of Kevlar’s strengths is due to hydrogen bonds between C=O and N-H groups in neighboring polymer chains (Figure 6). Kevlar derives additional strength from the interaction between unhybridized p orbitals (in the benzene ring as well as on the N, C, and O atoms) in one layer (see structure of a single layer below) with unhybridized p orbitals in the layers above and below it. This intermolecular force is called aromatic stacking.
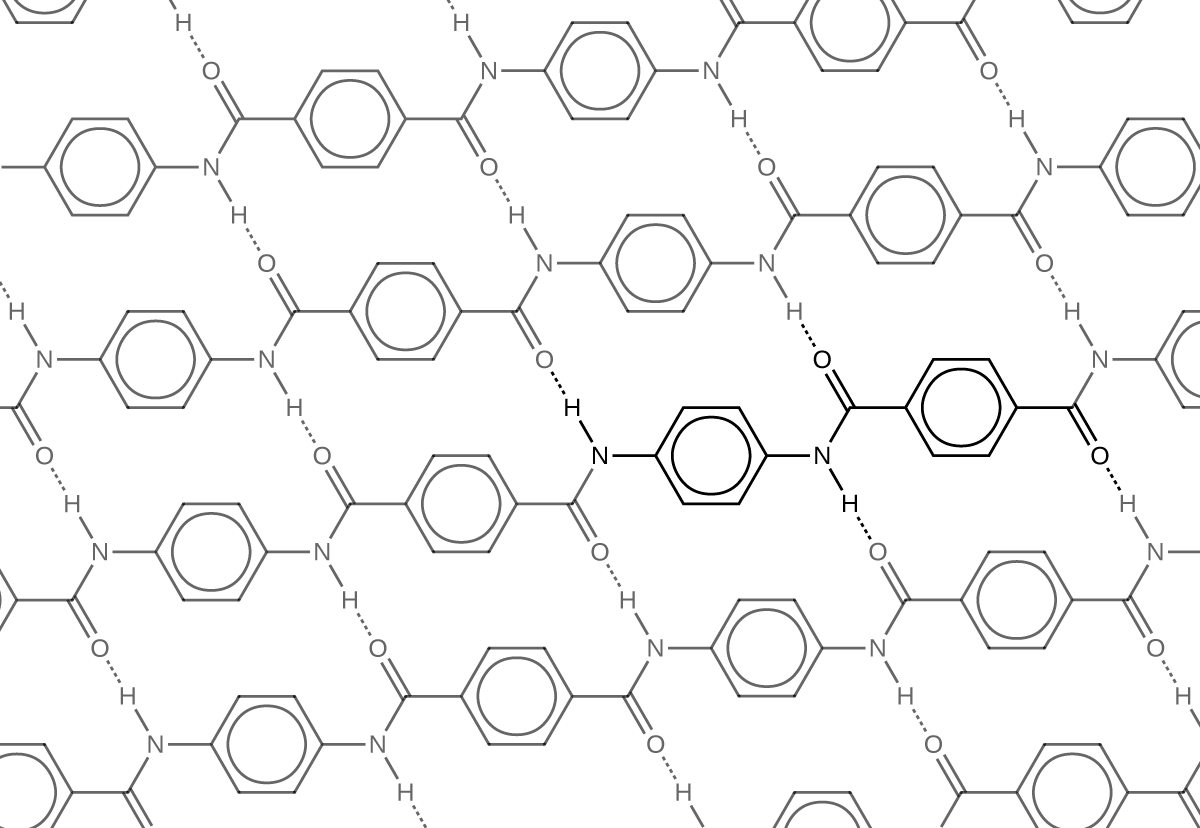
In addition to its better-known uses, Kevlar is used in cryogenics because of its very low thermal conductivity. Kevlar maintains its high strength when cooled to the temperature of liquid nitrogen (–196 °C). Many other plastics become brittle and break.
D16.5 Condensation Polymers: Proteins
An online tutorial on proteins is available so this section consists solely of this link: Protein tutorial 1 module. It is important that you work through Protein tutorial 1 now. If the tutorial is not formatted nicely, try resizing the window.
Exercise 2: Amino Acids in a Tripeptide
Podia Question
Answer the questions below. Table 1 may be useful in formulating answers. The repeating unit of nylon-6,10 has this structure.
1. The strength or toughness of a polymeric material depends on strengths of intermolecular forces between polymer chains and several other factors. Assuming other factors are equal, compare the toughness/strength of polyethylene and polyacrylonitrile. Explain clearly which is stronger and why.
2. Write the structures of the two monomers that can be combined to form nylon-6,10.
3. Name all intermolecular forces that attract polymer chains in nylon-6,10.
4. Depending on the orientation of adjacent polymer chains, intermolecular forces between nylon-6,10 chains might be stronger or weaker. Draw structures of two nylon-6,10 repeating units, one above and one below the structure given. Orient the structures you draw to maximize intermolecular forces.
5. Indicate in your drawing which types of intermolecular forces are important between which parts of the molecules. Also, write an explanation in words telling which forces are important where and why.
Two days before the next whole-class session, this Podia question will become live on Podia, where you can submit your answer.