Unit One
Day 7: Covalent Molecular Substances and Lewis Structures
As you work through this section, if you find that you need a bit more background material to help you understand the topics at hand, you can consult “Chemistry: The Molecular Science” (5th ed. Moore and Stanitski) Chapter 6-2 through 6-4 and Chapter 2-7, and/or Chapter 4.5-4.7 in the Additional Reading Materials section.
D7.1 Covalent Molecular Substances
A covalent molecular substance consists of molecules. A molecule is a group of atoms held together by covalent bonding. Examples are water, sucrose (table sugar), carbon dioxide (dry ice), and iodine (See Figure 1).
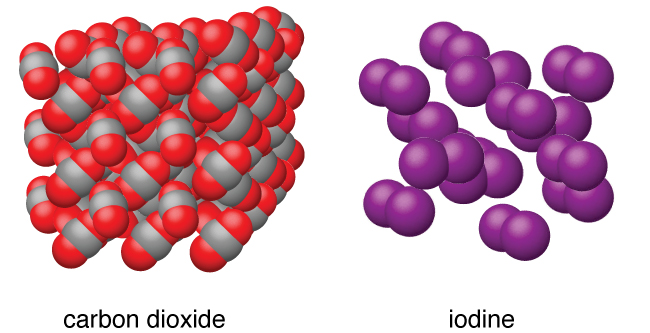
Activity 1: Reflection
Covalent molecular substances have properties that are significantly different from the properties of metals (Day 4, Section 6) or ionic compounds (Day 5, Section 6) and therefore it is useful to be able to classify a substance within one of these three categories. Typically, a covalent molecular substance consists of non-metals or metalloids—elements from the upper right of the periodic table (excepting noble gases but including H). Whereas metals and ionic compounds are usually solids at room temperature, many covalent molecular substances are liquids or gases; that is, they melt (and some boil) below room temperature or not too far above. Covalent molecular substances do not conduct electricity well as solids or liquids, the solids are weak and brittle or soft and waxy, and many are insoluble in water.
Activity 2: Types of Substances
In your notebook, make a table that lists characteristic properties of covalent molecular substances, ionic compounds, and metals. Include enough properties so that another student could use the table to distinguish each kind of substance based on a list of the properties. When you complete your table, click here to see an example table.
For each type of substance, write in your course notebook an explanation for each property based on atomic-level structure.
In a molecule, atoms are connected by covalent bonds that result from overlap of atomic orbitals. There are several important aspects of molecular structure:
- What kinds of atoms and how many of each are in the molecule?
- Which atoms are bonded to which other atoms?
- What are the distances between atoms that result in lowest energy? (Day 6, Section 1, Figure 4 shows the distance between two H atoms where energy is lowest—the bond length.)
- How strong are the bonds?
- What are the angles between the bonds?
- What is the arrangement of the atoms in three-dimensional space?
These factors determine molecular structure; molecular structure determines the properties of covalent molecular substances.
For example, in a water molecule the two H and one O atom are arranged with the H atoms each bonded to the O atom. The bonds are strong—separating the atoms is difficult. The angle between the two O–H bonds is 104.5°, somewhat more than a right angle. Angles larger or smaller than 104.5° result in higher energy (lower stability). The properties of water would be quite different if the three atoms were all on a line (bond angle of 180°).
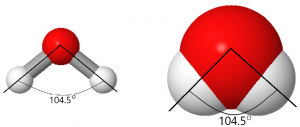
Exercise 1: Classifying Substances
D7.2 Structures of Covalent Molecules
As we noted at the end of Day 6, molecular orbitals for molecules with three or more atoms are complicated and hard to draw. Thus, although MOs would convey a more descriptive and accurate picture of electron distribution within a molecule, chemists often rely on simpler diagrams to depict the atoms and bonds. Keep in mind, however, that there are aspects of molecular structure that such simpler diagrams do not represent.
Activity 3: Lewis Diagrams
Consider each element listed below. In your course notebook write the electron configuration for an atom of each element, determine the number of valence electrons, and write a Lewis diagram (see section D4.4). How are the Lewis diagrams related to the position of each element in the periodic table?
N C S As O Br F Si H
Lewis diagrams for the constituent atoms can be combined to make a Lewis structure for a molecule. A Lewis structure shows each single bond in a molecule as a pair of electrons shared between two adjacent atoms. Valence electrons that are not in a bond are shown as dots associated with individual atoms. Here are Lewis diagrams for two chlorine atoms and a Lewis structure for a Cl2 molecule:
In Cl2 each Cl atom has three lone pairs (unshared electrons not used in bonding ) and shares one pair of electrons with the other Cl atom, forming a bond pair. Hence, each Cl atom is surrounded by eight valence electrons. Instead of a pair of dots, a bond pair is typically represented by a line—a single bond:
Activity 4: Lewis Structure and Electron Sharing
The Octet Rule
The term octet rule refers to the tendency of atoms of main-group elements to gain, lose, or share enough electrons to form an octet: eight valence electrons (a noble gas electron configuration).
The Lewis diagram for an atom can be used to predict the number of bonds the atom will form. For example, a carbon atom has four valence electrons and therefore requires four more electrons to reach an octet:
Because a hydrogen atom needs only two electrons to fill its valence shell, H is an exception to the octet rule and forms only one bond. The transition elements also do not follow the octet rule.
Exercise 2: Number of Bonds
Double and Triple Bonds
Two atoms may need to share more than one pair of electrons to achieve the requisite octet. A double bond consists of two pairs of electrons are shared between two atoms. For example:
A triple bond forms when three pairs of electron are shared between two atoms, as in carbon monoxide and the cyanide ion:
Activity 5: Double and Triple Bonds
Write answers to these questions in your course notebook:
Write a Lewis structure for N2 and a Lewis structure for O2. Describe the type of bond in each case.
Do the N atoms in N2 and the O atoms in O2 follow the rule for number of bonds in Exercise 2?
Use the molecular-orbital energy-level diagram in Day 6 to calculate the bond order for N2 and for O2. How do the bond orders relate to the Lewis structures?
D7.3 Writing Lewis Structures with the Octet Rule
Here is a step-by-step procedure for drawing Lewis structures of molecules and polyatomic ions:
- Determine the total number of valence electrons. For cations, subtract one electron for each positive charge. For anions, add one electron for each negative charge.
- Choose one or more central atoms; a central atom bonds to several other atoms and is usually the atom the forms the greatest number of bonds. (Usually the central atom is written first in a chemical formula, such as P in PCl3.) If there are two or more central atoms, connect them using single bond lines.
- Draw a skeleton structure of the molecule by arranging the other atoms (which are called terminal atoms) around the central atom(s). Connect terminal atoms to the central atom(s) by single bond lines.
- Distribute the remaining electrons as lone pairs on the terminal atoms (except hydrogen), completing an octet around each atom.
- If there are still valence electrons available, place them on the central atom(s).
- If the number of electrons around a central atom is less than an octet, rearrange the electrons to make multiple bonds with the central atom(s) until each atom has an octet.
Let’s apply these rules to a simple molecule, ammonia, NH3.
Here is a more complicated case: ethene (ethylene), C2H4.
Exercise 3: Lewis Structures and Valence Electrons
If you were to build a Lewis structure for nitrate ion, how many electrons would you need to allocate in your structure? (In other words, how many non-core electrons have to be in your structure?)
Exercise 4: Identifying Incorrect Lewis Structures
For each of the following Lewis structure, identify whether the structure is correct or not. If the structure is incorrect, identify the error made in the representation.
D7.4 Hydrocarbons: Alkanes
Typical properties of covalent molecular substances are exemplified by hydrocarbons, compounds that contain only the elements carbon and hydrogen. Hydrocarbon molecules can have a variety of shapes, which chemists usually depict using Lewis structures: long chains of C atoms connected by single bonds; chains with branches; chains folded back on themselves to form rings; chains, branched chains, or rings that include double or triple bonds. Many hydrocarbons are found in plants, animals, and their fossils; other hydrocarbons have been prepared in the laboratory. We use hydrocarbons every day, mainly as fuels, such as natural gas (mainly methane in the U.S.), acetylene, propane, butane, gasoline, diesel fuel, and heating oil. The familiar plastics polyethylene, polypropylene, and polystyrene are also hydrocarbons.
Exercise 5: Combustion of Octane
When one mole of octane (C8H18) burns in air, how many moles of carbon dioxide (CO2) will form? How many moles of water (H2O) will form?
The simplest hydrocarbons are alkanes, which contain only single covalent bonds between carbon atoms. Each of the carbon atoms in an alkane is bonded to four other atoms, each of which is either carbon or hydrogen. Noncyclic alkanes have the general formula CnH2n+2, where the number of H atoms is two more than twice the number of C atoms. Alkanes are also called saturated hydrocarbons, because each C atom is saturated—bonded to the maximum possible number of H atoms.
Lewis structures indicate atomic connectivity (which atoms are connected to which other atoms) but this does not indicate the molecular shape in three dimensions. In a Lewis structure the carbon atoms are usually along a straight lines and unbranched alkanes are often called “straight-chain” alkanes. However, as the ball-and-stick and space-filling models of pentane in Figure 2 show, the C atoms do not lie in a straight line. The carbon-carbon bond angle is actually about 109°.
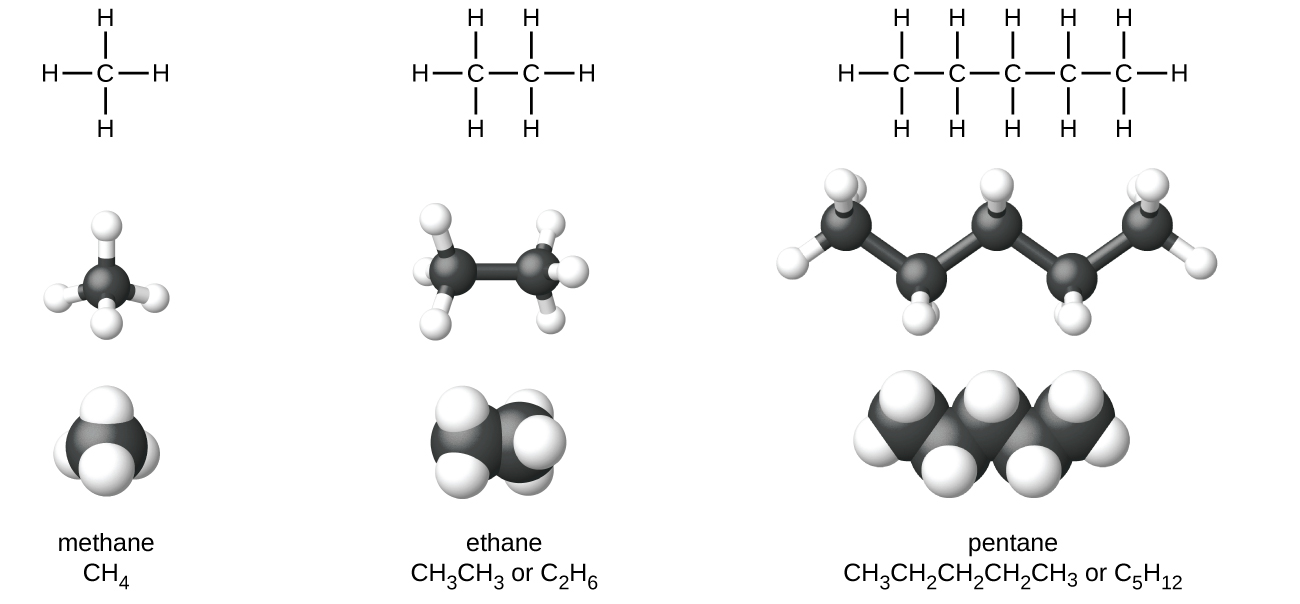
The structures of alkanes may also be represented by condensed structural formulas, such as CH3CH3, shown below the names in Figure 2. Condensed structural formulas indicate how many H atoms are bonded to each C atom; they are related to Lewis structures, but all the bond symbols have been removed.
Recall from Day 6, Section 2, that the molecular orbital of a σ covalent bond has cylindrical symmetry along the internuclear axis. This means that regardless of how two σ-bonded atoms are rotated around the internuclear axis, a σ bond can form between them and the molecule’s energy changes very little. Hence, the atom at one end of a single bond can rotate easily relative to the atom at the other end. Rotations around C–C bonds in an alkane produce different molecular shapes. The various molecular shapes are called conformational isomers (or conformers). They have the same molecular formula and same atomic connectivity. Because rotation around single bonds occurs readily at room temperature, we cannot isolate one conformer from another. Conformers are the same chemical compound, with the same name and the same physical properties.
D7.5 Constitutional Isomers
Compounds with the same molecular formula but different atomic connectivity are called constitutional isomers (or structural isomers). For example, there are two alkanes with the formula C4H10:
Activity 6: Analyzing Constitutional Isomers
In your course notebook write an answer to each question and an explanation of your answer:
Describe the shape of the n-butane molecule and how it differs from the shape of the 2-methylpropane molecule.
Analyze the bonding in each molecule. Are all C atoms bonded to the same number of H atoms? If not, is the number of similarly bonded C atoms the same in each of the two structures ?
Could you convert one structure to the other solely by rotating around one or more C–C bonds? To convert one structure to the other, would one or more chemical bonds need to be broken so that atoms could be re-arranged?
Would you expect the physical properties (such as melting point and boiling point) to be different for the two substances?
The n– in n-butane stands for normal, an unbranched carbon chain. Typically the n– is omitted and the compound is just named “butane”; it is assumed that you know that no prefix refers to the unbranched chain. Because it is an isomer of butane, 2-methylpropane is sometimes called isobutane.
To change one constitutional isomer to another requires breaking and re-forming chemical bonds; breaking bonds requires significant input of energy and at room temperature very few molecules have that much energy. Therefore, structural isomers can be synthesized and separated from one another: they are different substances.
Because Lewis structures are not intended to indicate the 3D geometry, Lewis structures that look different may actually represent the same substance. For example, the three structures below all represent the same molecule, butane, and hence are not different isomers.
One way to tell whether a Lewis structure is the same as another is to work out the name of the compound. Names are designed so that the same structure always has the same name; if two structures are different, naming them correctly will result in different names. You will not be explicitly tested on naming compounds (nomenclature) in this course, but it is useful to know how to name common molecules that you will encounter. You can read more about alkane nomenclature in the appendix, as well as Appendix E in “Chemistry: The Molecular Science” (5th ed. Moore and Stanitski).
Exercise 6: Naming Alkanes
Activity 7: Wrap-up
Podia Question
Compare each Lewis structure to the initial structure. Classify each structure as the same as the initial structure, an isomer of the initial structure, or neither the same as nor an isomer of the initial structure. Explain your reasoning using scientifically appropriate language.
Two days before the next whole-class session, this Podia question will become live on Podia, where you can submit your answer.