M2Q5: Monatomic Ions
Introduction
This section explores monatomic ions and naming simple compounds in greater detail. The section below provides more detailed description of these topics, worked examples, practice problems and a glossary of important terms.
Learning Objectives for Monatomic Ions
- Recognize that atoms bond to other atoms to form molecules and ions.
| Properties of Ionic Compounds | Properties of Covalent Compounds - Know the names and symbols of certain elements. Write formulas for ionic compounds.
| Simple Ionic Compounds | Naming Ionic Compounds |
- Apply Coulomb’s Law to rank melting points of different ionic substances.
| Coulomb’s Law |
| Key Concepts and Summary | Key Equations | Glossary | End of Section Exercises |
Ionic vs. Covalent Compounds
Properties of Ionic Compounds
When an element composed of atoms that readily lose electrons (a metal) reacts with an element composed of atoms that readily gain electrons (a nonmetal), a transfer of electrons usually occurs, producing ions. The compound formed by this transfer is stabilized by the electrostatic attractions (ionic bonds) between the ions of opposite charge present in the compound. A compound that contains ions and is held together by ionic bonds is called an ionic compound.
For example, when each sodium atom in a sample of sodium metal (group 1) gives up one electron to form a sodium cation, Na+, and each chlorine atom in a sample of chlorine gas (group 17) accepts one electron to form a chloride anion, Cl−, the resulting compound is NaCl (sodium chloride). This compound is composed of sodium ions and chloride ions in the ratio of one Na+ ion for each Cl− ion. Similarly, each calcium atom (group 2) can give up two electrons and transfer one to each of two chlorine atoms to form CaCl2, which is composed of Ca2+ and Cl− ions in the ratio of one Ca2+ ion (indicated by the inferred “1” subscript in the formula) to two Cl− ions (indicated by the subscript “2” in the formula).
In every ionic compound, the total positive charges equals the total negative charges—ionic compounds are electrically neutral overall, even though they contain positive and negative ions. We can use this observation to help us write the formula of an ionic compound, which must have a ratio of ions such that the numbers of positive and negative charges are equal.
You can often recognize ionic compounds because of their properties. Ionic compounds are typically solids at room temperature, and melt at high temperatures and boil at even higher temperatures. For example, sodium chloride melts at 801 °C and boils at 1413 °C. (As a comparison, water, a molecular compound, melts at 0 °C and boils at 100 °C.) In solid form, an ionic compound is not electrically conductive because its ions are unable to flow (“electricity” is the flow of charged particles). When molten, however, it can conduct electricity because its ions are able to move freely through the liquid (Figure 1).
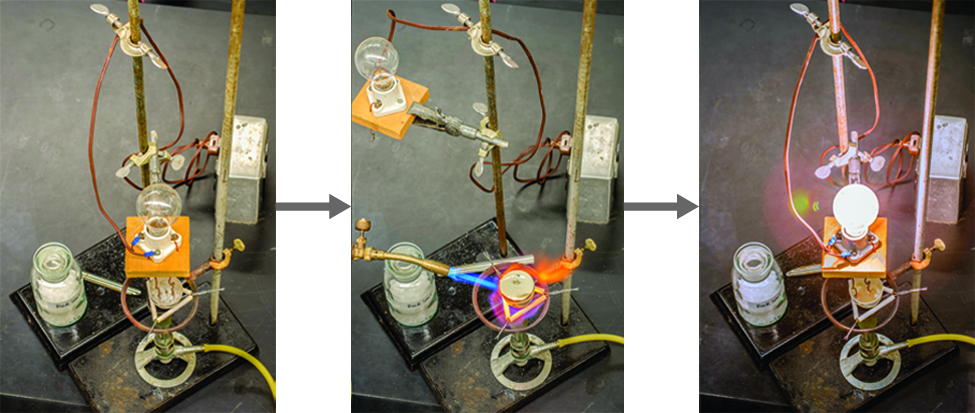
Watch this video to see a mixture of salts melt and conduct electricity.
Properties of Covalent Compounds
Many compounds do not contain ions but instead consist solely of discrete, neutral molecules. These covalent compounds (or molecular compounds) result when atoms share, rather than transfer (gain or lose), electrons. Covalent bonding is an important and extensive concept in chemistry, and it will be treated in considerable detail in a later module on chemical bonding.
We can often identify covalent compounds on the basis of their physical properties. Under normal conditions, covalent compounds often exist as gases, low-boiling-temperature liquids, and low-melting-temperature solids, although many important exceptions exist.
Whereas ionic compounds are usually formed when a metal and a nonmetal combine, covalent compounds are usually formed by a combination of nonmetals. Thus, the periodic table can help us recognize many of the compounds that are covalent. While we can use the positions of a compound’s elements in the periodic table to predict whether it is ionic or covalent at this point in our study of chemistry, you should be aware that this is a very simplistic approach that does not account for a number of interesting exceptions. Shades of gray exist between ionic and covalent compounds, and you’ll learn more about those later.
Example 1
Predicting the Type of Bonding in Compounds
Predict whether the following compounds are ionic or molecular:
- KI, the compound used as a source of iodine in table salt
- H2O2, the bleach and disinfectant hydrogen peroxide
- CHCl3, the anesthetic chloroform
- Li3N, the only stable alkali metal nitride
Solution
- Potassium (group 1) is a metal, and iodine (group 17) is a nonmetal; KI is predicted to be ionic.
- Hydrogen (group 1) is a nonmetal, and oxygen (group 16) is a nonmetal; H2O2 is predicted to be molecular.
- Carbon (group 14) is a nonmetal, hydrogen (group 1) is a nonmetal, and chlorine (group 17) is a nonmetal; CHCl3 is predicted to be molecular.
- Lithium (group 1) is a metal, and nitrogen (group 15) is a nonmetal; Li3N is predicted to be ionic.
Check Your Learning
Using the periodic table, predict whether the following compounds are ionic or covalent:
- SO2
- CaF2
- N2H4
- Al2O3
Answer:
(a) covalent; (b) ionic; (c) covalent; (d) ionic
Simple Ionic Compounds
In ordinary chemical reactions, the nucleus of each atom (and thus the identity of the element) remains unchanged. Electrons, however, can be added to atoms by transfer from other atoms, lost by transfer to other atoms, or shared with other atoms. The transfer and sharing of electrons among atoms govern the chemistry of the elements. During the formation of some compounds, atoms gain or lose electrons, and form electrically charged particles called ions (Figure 2).
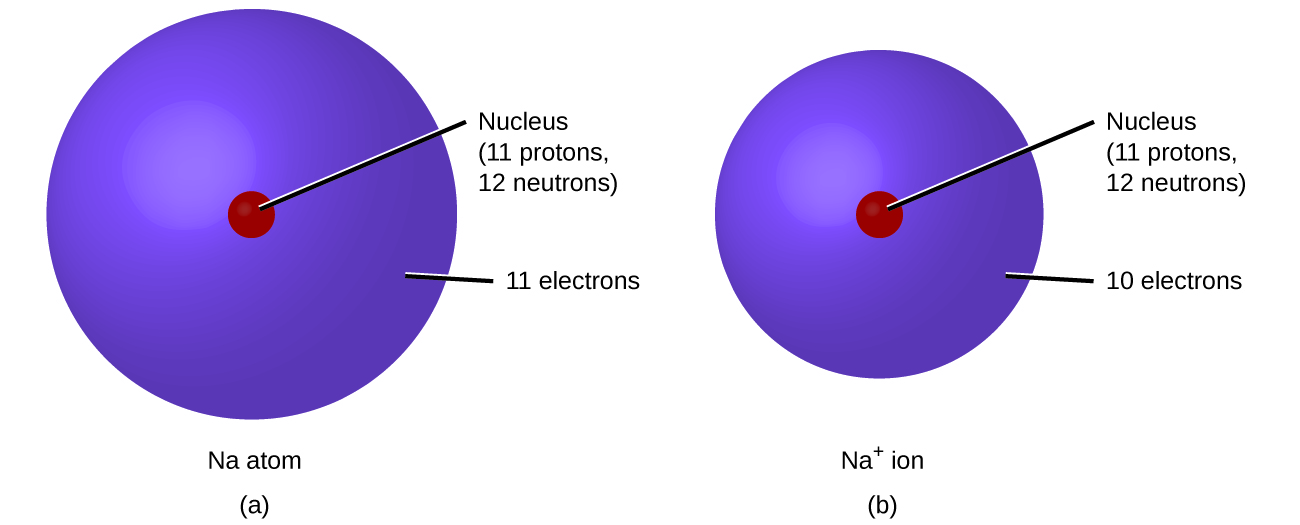
You can use the periodic table to predict whether an atom will form an anion or a cation, and you can often predict the charge of the resulting ion. Atoms of many main-group metals lose enough electrons to leave them with the same number of electrons as an atom of the preceding noble gas. In other words, an atom of an alkali metal (group 1) loses one electron and forms a cation with a 1+ charge; an alkaline earth metal (group 2) loses two electrons and forms a cation with a 2+ charge, and so on. For example, a neutral calcium atom, with 20 protons and 20 electrons, readily loses two electrons. This results in a cation with 20 protons, 18 electrons, and a 2+ charge. It is symbolized Ca2+, and has the same number of electrons as atoms of the preceding noble gas, argon. The name of a metal ion is the same as the name of the metal atom from which it forms, so Ca2+ is called a calcium ion.
When atoms of nonmetal elements form ions, they generally gain enough electrons to give them the same number of electrons as an atom of the next noble gas in the periodic table. Atoms of group 17 gain one electron and form anions with a 1− charge; atoms of group 16 gain two electrons and form ions with a 2− charge, and so on. For example, the neutral bromine atom, with 35 protons and 35 electrons, can gain one electron. This results in an anion with 35 protons, 36 electrons, and a 1− charge. It is symbolized Br−, and has the same number of electrons as atoms of the next noble gas, krypton. (A discussion of the theory supporting the favored status of noble gas electron numbers reflected in these predictive rules for ion formation is provided in a later module on chemical bonding.)
Note the usefulness of the periodic table in predicting likely ion formation and charge (Figure 3). Moving from the far left to the right on the periodic table, main-group elements tend to form cations with a charge equal to the group number. That is, group 1 elements form 1+ ions; group 2 elements form 2+ ions, and so on. Moving from the far right to the left on the periodic table, elements often form anions with a negative charge equal to the number of groups moved left from the noble gases. For example, group 17 elements (one group left of the noble gases) form 1− ions; group 16 elements (two groups left) form 2− ions, and so on. This trend can be used as a guide in many cases, but its predictive value decreases when moving toward the center of the periodic table. In fact, transition metals and some other metals often exhibit variable charges that are not predictable by their location in the table. For example, copper can form ions with a 1+ or 2+ charge, and iron can form ions with a 2+ or 3+ charge.
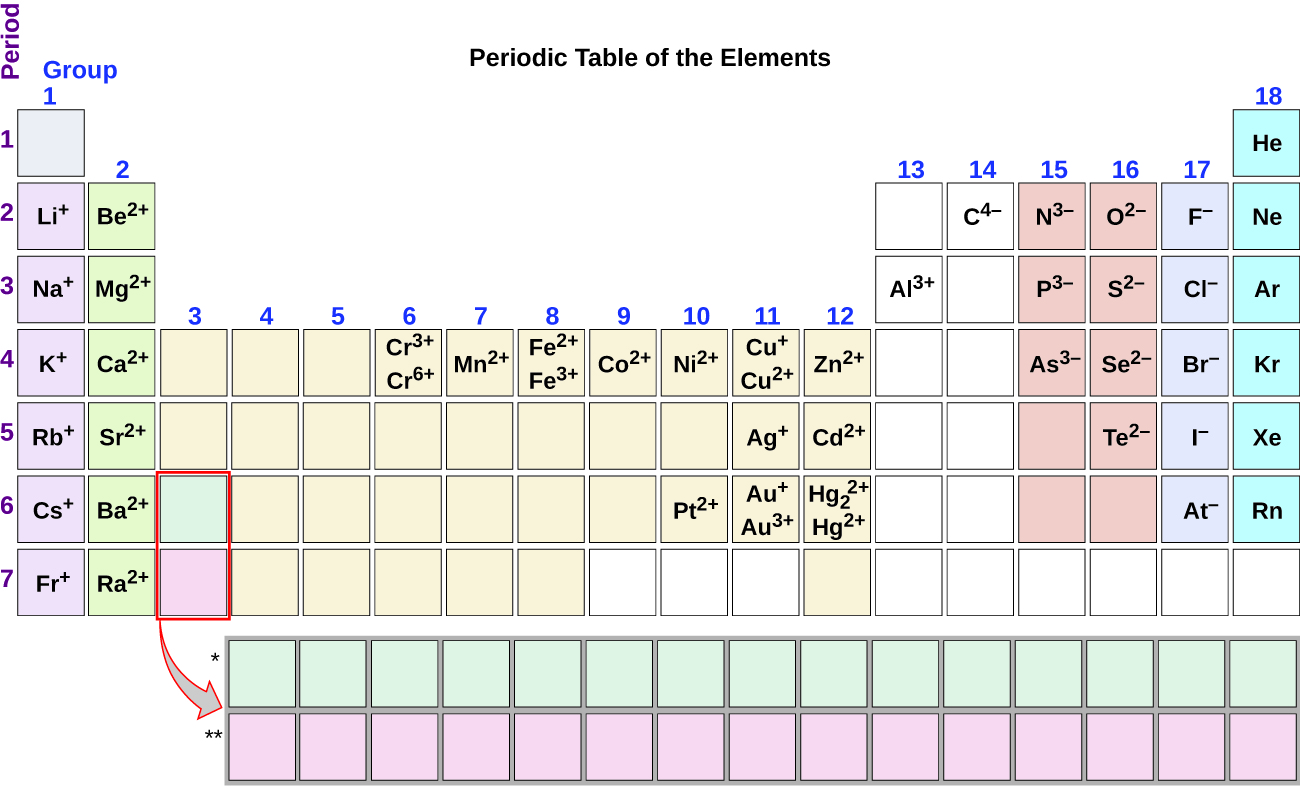
Example 2
Composition of Ions
An ion found in some compounds used as antiperspirants contains 13 protons and 10 electrons. What is its symbol?
Solution
Because the number of protons remains unchanged when an atom forms an ion, the atomic number of the element must be 13. Knowing this lets us use the periodic table to identify the element as Al (aluminum). The Al atom has lost three electrons and thus has three more positive charges (13) than it has electrons (10). This is the aluminum cation, Al3+.
Check Your Learning
Give the symbol and name for the ion with 34 protons and 36 electrons.
Answer:
Se2−, the selenide ion
Example 3
Formation of Ions
Magnesium and nitrogen react to form an ionic compound. Predict which forms an anion, which forms a cation, and the charges of each ion. Write the symbol for each ion and name them.
Solution
Magnesium’s position in the periodic table (group 2) tells us that it is a metal. Metals form positive ions (cations). A magnesium atom must lose two electrons to have the same number electrons as an atom of the previous noble gas, neon. Thus, a magnesium atom will form a cation with two fewer electrons than protons and a charge of 2+. The symbol for the ion is Mg2+, and it is called a magnesium ion.
Nitrogen’s position in the periodic table (group 15) reveals that it is a nonmetal. Nonmetals form negative ions (anions). A nitrogen atom must gain three electrons to have the same number of electrons as an atom of the following noble gas, neon. Thus, a nitrogen atom will form an anion with three more electrons than protons and a charge of 3−. The symbol for the ion is N3−, and it is called a nitride ion.
Check Your Learning
Aluminum and carbon react to form an ionic compound. Predict which forms an anion, which forms a cation, and the charges of each ion. Write the symbol for each ion and name them.
Answer:
Al will form a cation with a charge of 3+: Al3+, an aluminum ion. Carbon will form an anion with a charge of 4−: C4−, a carbide ion.
Example 4
Predicting the Formula of an Ionic Compound
The gemstone sapphire (Figure 4) is mostly a compound of aluminum and oxygen that contains aluminum cations, Al3+, and oxygen anions, O2−. What is the formula of this compound?
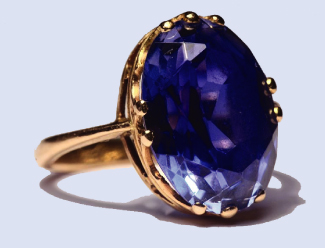
Solution
Because the ionic compound must be electrically neutral, it must have the same number of positive and negative charges. Two aluminum ions, each with a charge of 3+, would give us six positive charges, and three oxide ions, each with a charge of 2−, would give us six negative charges. The formula would be Al2O3.
Check Your Learning
Predict the formula of the ionic compound formed between the sodium cation, Na+, and the sulfide anion, S2−.
Answer:
Na2S
Because an ionic compound is not made up of discrete molecules, it may not be properly symbolized using a molecular formula. Instead, ionic compounds must be symbolized by a formula indicating the relative numbers of its constituent ions. For compounds containing only monatomic ions (such as NaCl), these formulas are just the empirical formulas (formula showing the composition of a compound given as the simplest whole-number ratio of atoms). We’ll revisit the crystal structure of salts in Module 11.
Naming Ionic Compounds
To name an inorganic compound, we need to consider the answers to several questions. First, is the compound ionic or covalent? If the compound is ionic, does the metal form ions of only one type (fixed charge) or more than one type (variable charge)? Are the ions monatomic or polyatomic? If the compound is covalent, does it contain hydrogen? If so, does it also contain oxygen? From the answers we derive, we place the compound in an appropriate category and then name it accordingly.
Compounds Containing Only Monatomic Ions
The name of a binary compound containing monatomic ions consists of the name of the cation (the name of the metal) followed by the name of the anion (the name of the nonmetallic element with its ending replaced by the suffix –ide). Some examples are given in Table 1.
NaCl, sodium chloride | Na2O, sodium oxide |
KBr, potassium bromide | CdS, cadmium sulfide |
CaI2, calcium iodide | Mg3N2, magnesium nitride |
CsF, cesium fluoride | Ca3P2, calcium phosphide |
LiCl, lithium chloride | Al4C3, aluminum carbide |
Compounds Containing a Metal Ion with a Variable Charge
Most of the transition metals can form two or more cations with different charges. Compounds of these metals with nonmetals are named with the same method as compounds in the first category, except the charge of the metal ion is specified by a Roman numeral in parentheses after the name of the metal. The charge of the metal ion is determined from the formula of the compound and the charge of the anion. For example, consider binary ionic compounds of iron and chlorine. Iron typically exhibits a charge of either 2+ or 3+ (Figure 3), and the two corresponding compound formulas are FeCl2 and FeCl3. The simplest name, “iron chloride,” will, in this case, be ambiguous, as it does not distinguish between these two compounds. In cases like this, the charge of the metal ion is included as a Roman numeral in parentheses immediately following the metal name. These two compounds are then unambiguously named iron(II) chloride and iron(III) chloride, respectively. Other examples are provided in Table 2.
Transition Metal Ionic Compound | Name |
FeCl3 | iron(III) chloride |
FeCl2 | iron(II) chloride |
Hg2O | mercury(I) oxide |
HgO | mercury(II) oxide |
Out-of-date nomenclature used the suffixes –ic and –ous to designate metals with higher and lower charges, respectively: Iron(III) chloride, FeCl3, was previously called ferric chloride, and iron(II) chloride, FeCl2, was known as ferrous chloride. Though this naming convention has been largely abandoned by the scientific community, it remains in use by some segments of industry. For example, you may see the words stannous fluoride on a tube of toothpaste. This represents the formula SnF2, which is more properly named tin(II) fluoride. The other fluoride of tin is SnF4, which was previously called stannic fluoride but is now named tin(IV) fluoride.
Example 5
Naming Ionic Compounds
Name the following ionic compounds, which contain a metal that can have more than one ionic charge:
- Fe2S3
- CuSe
- GaN
- CrCl3
Solution
The anions in these compounds have a fixed negative charge (S2−, Se2− , N3−, and Cl−), and the compounds must be neutral. Because the total number of positive charges in each compound must equal the total number of negative charges, the positive ions must be Fe3+, Cu2+, Ga3+, and Cr3+. These charges are used in the names of the metal ions:
- iron(III) sulfide
- copper(II) selenide
- gallium(III) nitride
- chromium(III) chloride
Check Your Learning
Write the formulas of the following ionic compounds:
- chromium(III) phosphide
- mercury(II) sulfide
- manganese(II) oxide
- copper(I) oxide
Answer:
(a) CrP; (b) HgS; (c) MnO; (d) Cu2O
Coulomb’s Law
At the submicroscopic level, ionic compounds are three dimensional ordered arrays of cations and anions held together by many strong ionic bonds. This array is often referred to as the crystalline lattice of the ionic compound (Figure 5). As such, most ionic compounds are solid materials at room temperature with relatively high melting points. Note that the chemical formula for an ionic compound indicates the smallest whole number ratio of the ions in the material but that even the tiniest crystal of an ionic compound contains many millions of ions. The structure and ion packing of crystalline lattices will be further explored in Module 11.
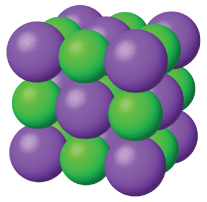
Insight into the strength of ionic bonds comes from an understanding of how charged entities such as cations and anions interact with each other. Unlike charges experience a force of attraction between them whereas like charges repel each other (Figure 6).
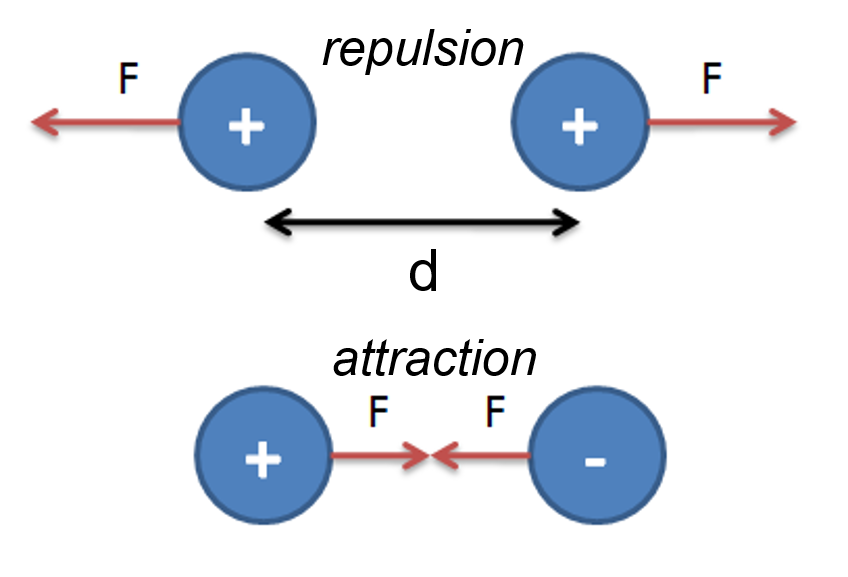
The magnitude of the force of attraction (or repulsion) is described by Coulomb’s Law:
Force of attraction = constant ×
Although we will not be using this equation to calculate actual numbers, this equation is very useful for predicting the relative strength of ionic bonds between different ions, and therefore the relative melting points for ionic compounds. The force of attraction is directly proportional to the magnitude of the charge on each ion, F ∝ Q1 and Q2, and therefore ionic compounds that consist of ions with higher charges will have higher melting points. For example, NaCl has a melting point of 801 °C, but MgO that consists of ions with a +2 and -2 charge respectively, has a melting point of 2825 °C. However, the size of the ions must also be taken into consideration because this determines the distance between them. Two smaller ions that are touching will have a shorter distance between their centers compared to two larger ions. The magnitude of the force is inversely proportional to the square of the distance between the two ions, F ∝ 1/d2. The smaller the ion the smaller this distance, and therefore the stronger the attractive force. The melting point of NaCl (801 °C) is higher than that for NaBr (747 °C), because the chloride ion is smaller than the bromide ion.
Example 6
Melting Point Comparisons
The precious gem ruby is aluminum oxide, Al2O3, containing traces of Cr3+. The compound Al2Se3 is used in the fabrication of some semiconductor devices. Which has the greater melting point, Al2O3 or Al2Se3?
Solution
In these two ionic compounds, the charges Q1 and Q2 are the same, so the difference in melting point will depend upon d. The O2– ion is smaller than the Se2– ion. Thus, Al2O3 would have a shorter interionic distance than Al2Se3, and Al2O3 would have the greater melting point.
Experiments support this prediction: the melting point of Al2O3 is 2072 °C, and the melting point of Al2Se3 is 947 °C.
Check Your Learning
Zinc oxide, ZnO, is a very effective sunscreen. How would the melting point of ZnO compare to that of NaCl?
Answer:
ZnO would have the greater melting point because the charges Q1 and Q2 are in ZnO are greater. The interionic distance of ZnO and NaCl are harder to predict based on your start-of-the-semester knowledge. Without knowing the interionic distance, one can generally apply the principle that the magnitude of the charge has a greater impact on melting point than the interionic distance.
*It turns out that the interionic distance of ZnO (214 pm) is smaller than NaCl (283 pm), and thus the melting point prediction follows Coulomb’s Law.
Experiments support this prediction: the melting point of ZnO is 1975 °C, and the melting point of NaCl is 801 °C.
Coulomb’s Law can also be stated in terms of energy or potential energy, U:
Potential Energy = U = constant ×
This looks very similar to Coulomb’s Law presented above, but the d in the denominator is not squared. Those of you familiar with physics may remember that work is a form of energy, and work is force × distance. For those of you familiar with calculus, the difference between force and energy is a result of force being the first derivative of the potential energy.
Using this form of the equation provides insight into the energy of interaction between two charged ions. As anions and cations are oppositely charged, Q1 and Q2 will have opposite signs, and U will therefore be more negative when charges are higher and distances between ions are smallest. This will result in a more stable, lower energy arrangement of the ions. When comparing ionic compounds in terms of their potential energy, the ones with the most negative U values (greater magnitude of U) will therefore have higher melting points. Whether Coulomb’s law is stated in terms of force or potential energy, the prediction that higher charges and smaller ions leads to ionic compounds with higher melting points is consistent.
The graph below depicts the relationship between the potential energy, U, and distance, d, between two ions (Figure 7).
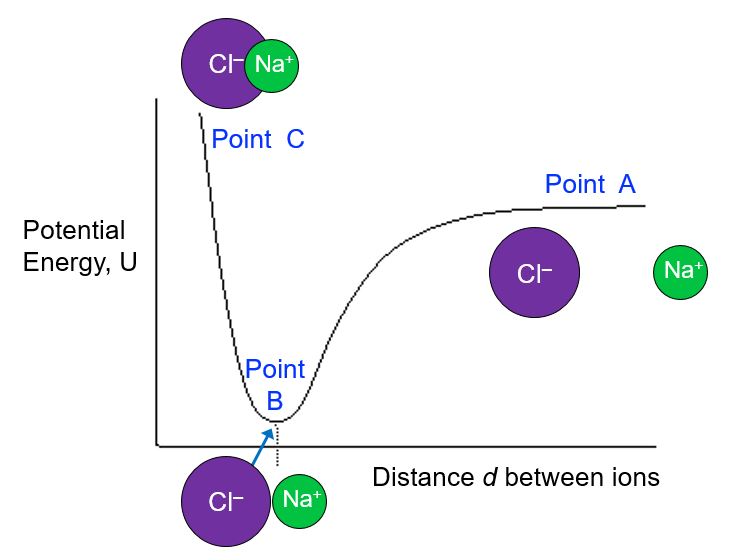
This type of plot is fundamental in chemistry and you will encounter it again when exploring covalent bonds in molecules. At Point A the two ions are relatively far apart and hardly interacting, but as they come closer together (moving to the left) the potential energy drops until it hits an energy minimum at Point B. Point B is the place where the ion pair is most stable as it occurs at the lowest energy. The distance at this point is the bond length of the ionic bond. The plot sharply increases as the distance between the two ions gets even closer indicating that there is now repulsion between the two ions. This is a result of the overlap of the electron clouds of the two ions, and even the repulsion of the nuclei of the two ions, resulting in a very unstable ion pair at Point C.
Key Concepts and Summary
Metals (particularly those in groups 1 and 2) tend to lose the number of electrons that would leave them with the same number of electrons as in the preceding noble gas in the periodic table, forming positively charged ions. Similarly, nonmetals (especially those in groups 16 and 17, and, to a lesser extent, those in Group 15) can gain the number of electrons needed to provide atoms with the same number of electrons as in the next noble gas in the periodic table. Thus, nonmetals tend to form negative ions. Positively charged ions are called cations, and negatively charged ions are called anions. Ions can be either monatomic (containing only one atom) or polyatomic (containing more than one atom, which we will discuss in the section on polyatomic ions).
Compounds that contain ions are called ionic compounds. Ionic compounds generally form from metals and nonmetals. Compounds that do not contain ions, but instead consist of atoms bonded tightly together in molecules (uncharged groups of atoms that behave as a single unit), are called covalent compounds. Covalent compounds usually form from nonmetals.
Binary ionic compounds typically consist of a metal and a nonmetal. The name of the metal is written first, followed by the name of the nonmetal with its ending changed to –ide. For example, K2O is called potassium oxide. If the metal can form ions with different charges, a Roman numeral in parentheses follows the name of the metal to specify its charge. Thus, FeCl2 is iron(II) chloride and FeCl3 is iron(III) chloride.
Key Equations
- Force of attraction = constant ×
- Potential Energy = U = constant ×
Glossary
- covalent bond
- attractive force between the nuclei of a molecule’s atoms and pairs of electrons between the atoms
- covalent compound
- (also, molecular compound) composed of molecules formed by atoms of two or more different elements
- ionic bond
- electrostatic forces of attraction between the oppositely charged ions of an ionic compound
- ionic compound
- compound composed of cations and anions combined in ratios, yielding an electrically neutral substance
- molecular compound
- (also, covalent compound) composed of molecules formed by atoms of two or more different elements
- monatomic ion
- ion composed of a single atom
- polyatomic ion
- ion composed of more than one atom
Chemistry End of Section Exercises
Ionic vs. Covalent Compounds
- Using the periodic table, predict whether the following chlorides are ionic or covalent: SiCl4, PCl3, CaCl2, CsCl, CuCl2, and CrCl3.
- For each of the following compounds, state whether it is ionic or covalent. If it is ionic, write the symbols for the ions involved:
- NF3
- BaO
- IBr
- Na2O
- H2S
- Ag2S
- N2Cl4
- For each of the following pairs of ions, write the symbol for the formula of the compound they will form:
- Ca2+, S2−
- Al3+, Br−
- K+, O2−
- Al3+, O2−
- True or False: Melting solid NaCl to form a molten liquid is a physical change.
- True or False: Mixing Ne and He gases is a chemical change.
Naming Ionic Compounds
- Write the formulas of the following compounds:
- rubidium bromide
- magnesium selenide
- sodium oxide
- calcium chloride
- hydrogen fluoride
- gallium phosphide
- aluminum bromide
- Write the formulas of the following compounds:
- titanium (IV) oxide
- titanium (II) oxide
- copper (I) chloride
- copper (II) chloride
- iron (II) oxide
- iron (III) oxide
Applying Coulomb’s Law
- We have used Coulomb’s Law
to rationalize relative melting points of certain salts.
- State the general trends about how magnitude of attraction between ions (as predicted by Coulomb’s Law) varies with respect to ion charge and ionic radius.
- Explain how the magnitude of attraction between ions (as predicted by Coulomb’s Law) connects to the melting point temperature. Be specific about what’s happening at the particle level.
- The graph below shows a plot of U versus r for two salts, MgO (melting point = 2885 °C) and BaO (melting point = 1923 °C). Identify which curve corresponds to which salt.
- True or False: MgCl2 has a higher melting point than BaCl2 because the force of attraction between Mg2+ and Cl– ions is greater than the force of attraction between Ba2+ and Cl– ions. (The diameters of Ba2+ and Mg2+ are 135 pm and 72 pm, respectively.)
- Place the following salts in order from lowest melting point to highest melting point based on predictions made using Coulomb’s Law: MgO, SrO, KF, CsF, MgF2.
- Place the following salts in order from lowest melting point to highest melting point based on predictions made using Coulomb’s Law: K2S, K2O, CaS, Cs2S, CaO.
- Which compound in each of the following pairs has the greater melting point? (Note: Ba2+ and K+ have similar radii; S2– and Cl– have similar radii.) Explain your choices.
- K2O or Na2O
- K2S or BaS
- KCl or BaS
- BaS or BaCl2
- Select the salt with the highest predicted melting point. (Radius of various ions are: Mg2+ = 86 pm; Na+ = 116 pm; Br– = 181 pm; F– = 119 pm; O2- = 126 pm)
- MgO
- Na2O
- NaBr
- NaF
- Fill in the table below with the formula of the salt formed between each ion pair. Which salt from your table would be predicted to have the lowest melting point?
Y– (133 pm radius) Z2- (140 pm radius) W2+ (72 pm radius) X+ (102 pm radius)
Answers to Chemistry End of Section Exercises
- Ionic: CaCl2, CsCl, CuCl2, CrCl3; Covalent: PCl3, SiCl4
- (a) covalent
(b) ionic (Ba2+ and O2-)
(c) covalent
(d) ionic (Na+ and O2-)
(e) covalent
(f) ionic (Ag+ and S2-)
(g) covalent - (a) CaS; (b) AlBr3; (c) K2O; (d) Al2O3
- True
- False
- (a) RbBr; (b) MgSe; (c) Na2O; (d) CaCl2; (e) HF; (f) GaP; (g) AlBr3
- (a) TiO2; (b) TiO; (c) CuCl; (d) CuCl2; (e) FeO; (f) Fe2O3
- (a) The larger the ionic charge, the greater the magnitude of attraction between the ions. The smaller the distance between ions, the greater the magnitude of the attraction between ions.
(b) The greater the magnitude of attraction, the more energy needed to separate the ions upon melting and thus the higher the melting point.
(c) The solid line is MgO, the dashed line if BaO. - True
- CsF (703 °C) < KF (858 °C) < MgF2 (1263 °C) < SrO (2531 °C) < MgO (2825 °C)
Left-click here to watch Exercise 10 problem solving video.
- Cs2S < K2S < K2O < CaS < CaO
- (a) Na2O, Na radius is smaller than K radius;
(b) BaS, Ba and K have similar radii but Ba has a 2+ charge;
(c) BaS, Ba and S both have magnitude charges of 2;
(d) BaS, larger charge magnitude - A
- XY is likely to have the lowest melting point.
Y– (133 pm radius) Z2- (140 pm radius) W2+ (72 pm radius) WY2 WZ X+ (102 pm radius) XY X2Z
Please use this form to report any inconsistencies, errors, or other things you would like to change about this page. We appreciate your comments. 🙂