Module 9: Molecular Geometry
Background
We have examined the basic ideas of bonding, showing that atoms share electrons to form molecules with stable Lewis structures. In this module, you will learn about Valence Shell Electron Pair Repulsion Theory (VSEPR) and how it allows us to predict the shapes of molecules. You will also learn how Lewis structures, VSEPR, and atomic electron configurations do not adequately explain all experimental observations of molecular geometry. For this reason, we will consider additional theories of bonding in molecules (Valence Bond Theory and Molecular Orbital Theory) as alternatives to Lewis structures and VSEPR theory. For example, MO Theory correctly explains the observed magnetic behavior of N2 and O2. We know that electrons and magnetic behavior are related through electromagnetic fields. Both N2 and O2 have fairly similar Lewis structures that contain lone pairs of electrons.
Yet oxygen demonstrates very different magnetic behavior than nitrogen. We can pour liquid nitrogen through a magnetic field with no visible interactions, while liquid oxygen (shown in Figure 1) is attracted to the magnet and floats in the magnetic field. We need to understand the additional concepts of valence bond theory, orbital hybridization, and molecular orbital theory to understand these observations.
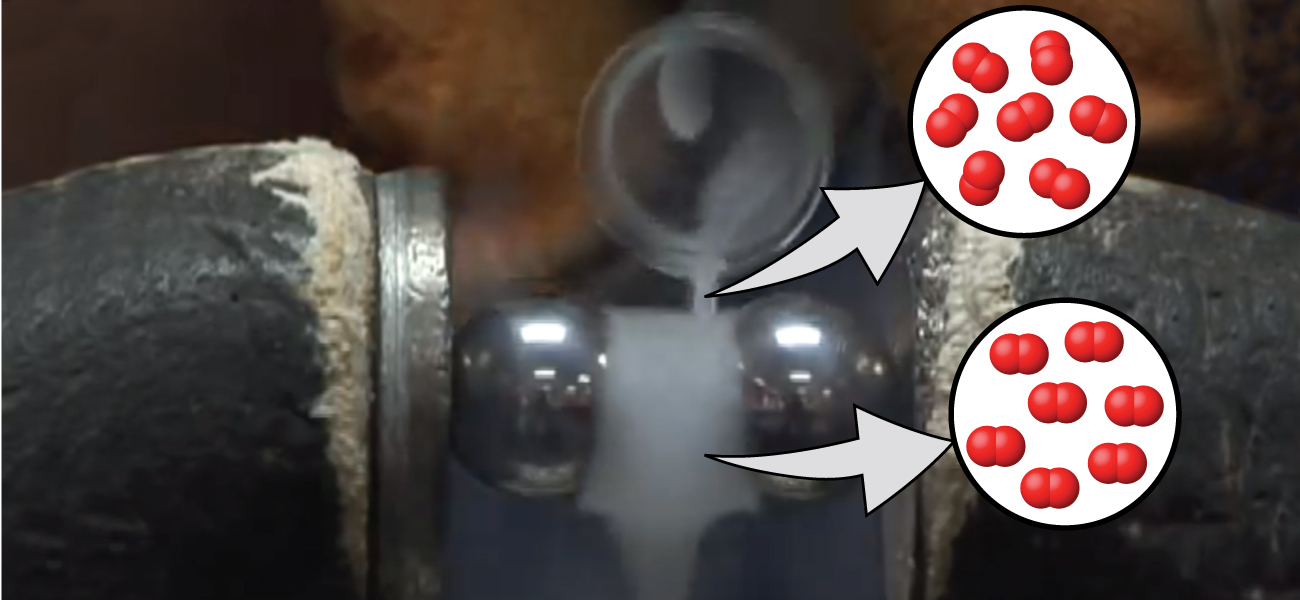
Learning Objectives for Molecular Geometry
- Predict molecular shape by applying Valence Shell Electron Pair Repulsion Theory (VSEPR).
- Draw and interpret three-dimensional representations of molecules using “dashed” and “wedge” bonds, and estimate bond angles.
- Predict molecular polarity and molecular dipole moments by considering molecular shape and bond polarity.
- Apply valence bond theory to predict orbital hybridization in atoms, to describe and illustrate sigma and pi bonds in molecules, and to rationalize cis/trans isomerization and/or resonance.
- Apply molecular orbital theory and interpret molecular orbital diagrams for diatomic molecules and ions.
Why is this content important?
In the news: Understanding the molecular structure of compounds has countless applications in our modern lives. From the discovery of new materials for electronics, such as semiconductors, to the implementation of new technologies to reduce our carbon footprints, such as solar cells, knowledge of molecular structure is important to many scientific and engineering areas of study. “Rational drug design” is one such field of study that is growing rapidly. Rational drug design is a term used to describe the process of designing and synthesizing therapeutic compounds (pharmaceutical drugs) based on the known structure of a specific target molecule. Whereas historical methods of finding and testing drugs were laborious, expensive, and potentially dangerous, modern computing techniques and understanding of molecular structure have revolutionized the methods behind finding cures for diseases. This article provides an example of how rational drug design uses a target molecule’s structure to guide the discovery of a cure.
Please use this form to report any inconsistencies, errors, or other things you would like to change about this page. We appreciate your comments. 🙂