D25.1 Acid Strength and Molecular Structure
Acid-base reactions, like many other chemical reactions, involve breaking and forming bonds. Hence, we can use our chemical understanding of molecular structure to understand what makes some acids stronger than others.
We know that an equilibrium favors the thermodynamically lower energy (more stable) side of the reaction, and that the magnitude of the equilibrium constant reflects the energy difference (ΔrG°) between the reactants and products. Consequently, for an acid-base equilibrium:
anything that lowers the energy of A– relative to HA, or raises the energy of HA relative to A–, will make ΔrG° more negative, increasing Ka, and hence make HA a stronger acid. Similarly, anything that lowers the energy of HA relative to A–, or raises the energy of A– relative to HA, will make ΔrG° more positive, decreasing Ka, and hence make HA a weaker acid. This idea is illustrated pictorially below.
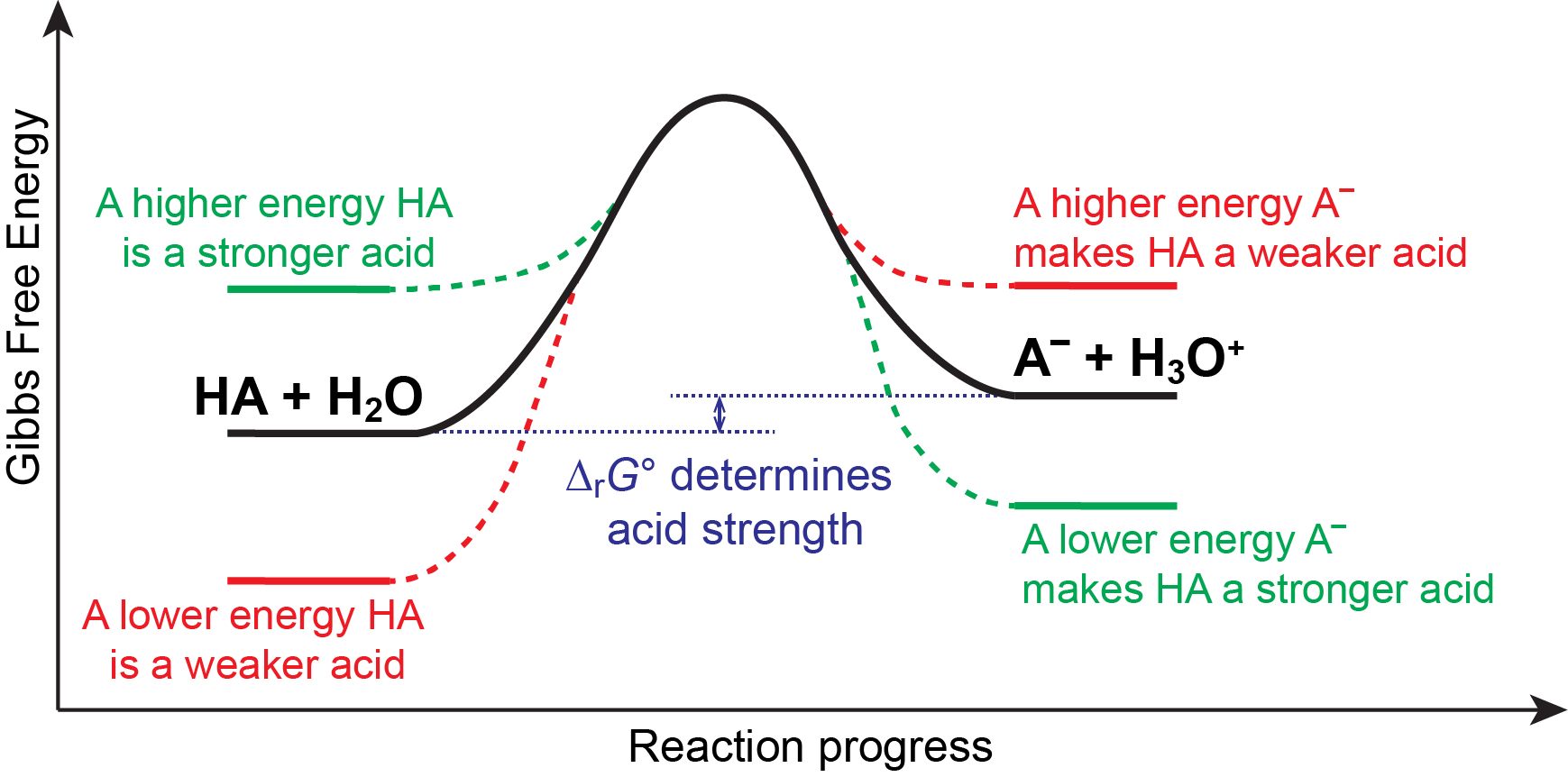
In other words, a strong acid is a molecule that is higher in energy (less stable) than its conjugate base. This instability relative to its conjugate base drives its reactivity in an acid-base reaction, making it a strong acid. Similarly, a weak acid is a molecule that is lower in energy (more stable) than its conjugate base. This relative stability makes it less reactive in an acid-base reaction, making it a weak acid.
The same thermodynamic reasoning apply when considering the basicity of a generic base A– in the equilibrium:
So how do we think about what stabilizes, or destabilizes, a molecule? There are a few factors to consider.
Bond Strength
In general, a stronger H–A bond corresponds to a more stable HA molecule, which leads to a less acidic HA.
This effect is illustrated by the hydrogen halide series [HX(aq) + H2O(ℓ) ⇌ X–(aq) + H3O+(aq)]:
HF | HCl | HBr | HI | |
---|---|---|---|---|
Average Bond Enthalpy (kJ/mol) | 566 | 431 | 366 | 299 |
ΔrG° (kJ/mol, 25 °C) | +18 | −35 | −51 | −53 |
Ka (25 °C) | 6.8 × 10−4 | 1.2 × 106 | 7.9 × 108 | 2 × 109 |
Note that the “average bond enthalpy” values listed above are associated with a different bond breaking reaction than acid-base reactions. Specifically, a hydrogen atom is produced instead of a hydrogen ion:
Nonetheless, these values can be used to estimate the bond strengths. In the hydrogen halide series, the overlap between the H 1s orbital and the halogen atom (X) valence orbital decreases as you go down the periodic group, leading to weaker H-X covalent bonds as reflected by their average bond enthalpy values. If a HX molecule is held together by a weaker bond, then the molecule itself is less stable. Therefore, as you go from HF to HI, the HX bond is weaker, the HX molecule is less stable, and HX is more acidic.
A similar trend is observed going down other groups. For example, for group 16 [H2Y(aq) + H2O(ℓ) ⇌ HY–(aq) + H3O+(aq)]:
H2O | H2S | H2Se | H2Te | |
---|---|---|---|---|
Average Bond Enthalpy (kJ/mol) | 467 | 347 | 276 | 238 |
ΔrG° (kJ/mol, 25 °C) | +80 | +40 | +22 | +15 |
Ka (25 °C) | 1.0 × 10−14 | 1.0 × 10-7 | 1.3 × 10-4 | 2.5 × 10-3 |
Electronegativity of the atom where the excess electron resides
Often, the conjugate base of an acid is negatively charged. For these molecules, factors that stabilize the excess electron would stabilize the conjugate base, and thereby favor the dissociation of the acid and make it a stronger acid. One of these factors is the electonegativity of the atom where the excess electron resides.
For example, consider the relative acidity of the following acids [HnZ(aq) + H2O(ℓ) ⇌ Hn-1Z–(aq) + H3O+(aq)]:
Acid (HnZ) | CH4 | NH3 | H2O | HF |
---|---|---|---|---|
Conjugate base (Hn-1Z–) | CH3– | NH2– | OH– | F– |
ΔrG° (kJ/mol, 25 °C) | +300 | +200 | +80 | +18 |
Ka (25 °C) | 1 × 10−50 | 1 × 10-36 | 1.0 × 10-14 | 6.8 × 10−4 |
In all four of these conjugate bases, the excess electron resides mostly on the second row element (C, N, O, or F), where the electronegativity trend is F > O > N > C. The excess electron (which gives rise to the -1 charge) is at a lower energy (more stable) when it resides on a more electronegative atom. Therefore, F– can stabilize the extra electron better than OH–, which is in turn better than NH2–, which is better than CH3–. Consequently, HF can dissociate and form H+ and F– to a greater extent compared to H2O can form H+ and OH–, and so forth. And this is increasing acid strength from CH4 to HF is indeed the trend we see in the table above.
The same trend is predicted by analyzing the acids: as the electronegativity of Z in HnZ increases, the Z–H bond becomes more polar (with greater δ– on Z and greater δ+ on H), thus favoring dissociation to form Hn-1Z– and H+. (Note that this difference in bond polarity does not inform on bond strength differences. The average bond enthalpies (kJ/mol) are: C-H (416), N-H (391), O-H (467), F-H (566).)
Due to both the increasing stability of the conjugate base and the increasing polarization of the Z–H bond in the acid, acid strengths of binary hydrides generally increase as we go from left to right across a row of the periodic table.
Electron Delocalization
Let us consider two types of compounds, carboxylic acids (RCOOH) and alcohols (ROH), acting as an acid in an acid-base reaction. For both species, an O-H bond is broken during the acid-base reaction. For example, at 25 °C,
While acetic acids are not strong acids (Ka is <1), they nonetheless are stronger acids than alcohols by 10,000,000,000 times. Why should the presence of a carbonyl group adjacent to a hydroxyl group have such a profound effect on the acidity of the hydroxyl proton?
Both the carboxylic acid and its conjugate base, the carboxylate anion, are stabilized by having additional resonance structures that delocalize electron densities. However, the stabilization in carboxylate anion is much greater because the two major resonance structures have equal contributions to the resonance hybrid, and the excess electron (negative charge) is shared equally between the two highly-electronegative oxygen atoms. This stabilization of the conjugate base leads to a markedly increased acidity of carboxylic acids.
The Ka values of several carboxylic acids at 25 °C are shown below.
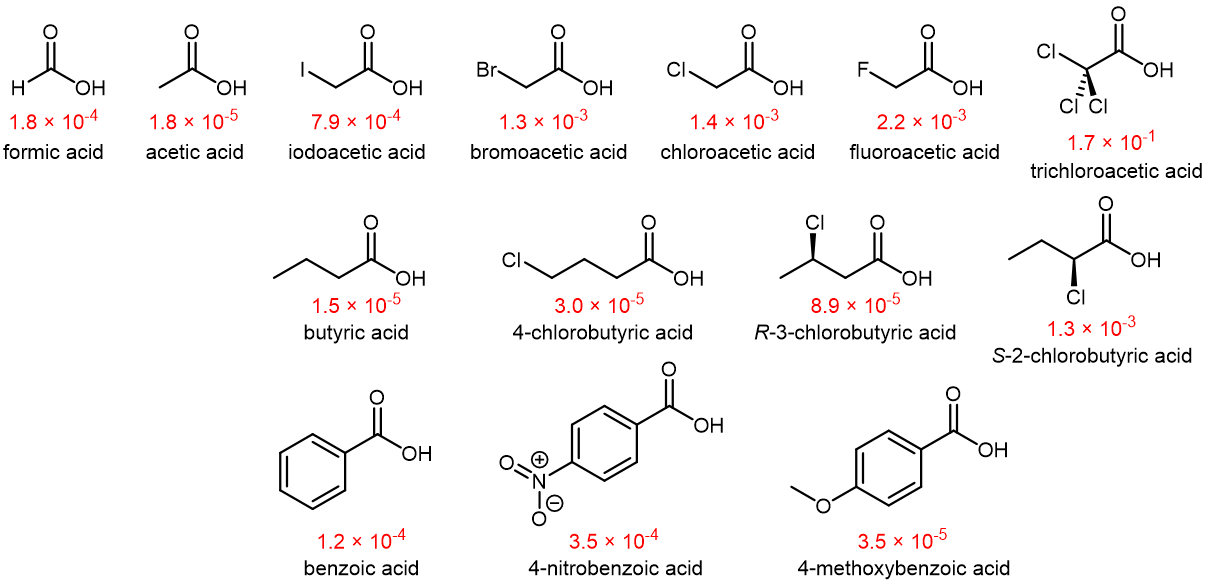
Inductive Effect
Atoms (or groups of atoms) in a molecule that are not directly bonded to the acidic H can also influence the molecule’s acidity. They can do so via an inductive effect, that is, they induce a polarization in the distribution of electrons within the molecule. This can be seen by studying the structures in Figure: Carboxylic Acids above. More electronegative substituents (F, Cl, Br) near the -COOH carboxyl group act to increase the acidity of the carboxylic acid. For example, fluoroacetic acid, CH2FCOOH, is significantly more acidic than acetic acid, CH3COOH.
This inductive effect works in two ways: It stabilizes the anionic conjugate base by drawing electron density away from the negatively charged -COO– group (it is another way for the excess electron to delocalize to more atoms within the molecule). It also polarizes and weakens the O-H bond in the -COOH group by drawing electron density away from this bond in the acid, thereby facilitating ionization of hydrogen as H+.
The magnitude of the inductive effect depends on the nature as well as the number of halogen substituents. For an example, consider several acetic acid derivatives:
CH3COOH | CH2ClCOOH | CH2FCOOH | CHCl2COOH | CCl3COOH | CF3COOH | |
ΔrG° (kJ/mol, 25 °C) | +27 | +16 | +15 | +7.7 | +4.4 | +3.0 |
Ka (25 °C) | 1.8 × 10−5 | 1.4 × 10−3 | 2.2 × 10−3 | 4.5 × 10−2 | 1.7 × 10−1 | 3.0 × 10−1 |
Fluorine, which is more electronegative than chlorine, causes a larger inductive effect as it draws away greater amount of electron density to itself. Having three halogens, as opposed to one or two, causes a larger inductive effect. Note that inductive effects can be quite significant. For instance, replacing the –CH3 group of acetic acid by a –CF3 group results in an almost 10,000-fold increase in acidity.
In another example, the acidity of hypohalous acids [HOX(aq) + H2O(ℓ) ⇌ OX–(aq) + H3O+(aq)] varies by about three orders of magnitude due to the difference in electronegativity of the halogen atoms:
ΔrG° (kJ/mol, 25 °C) | Ka (25 °C) | |
---|---|---|
HOCl | +41 | 6.8 × 10−8 |
HOBr | +50 | 3 × 10−9 |
HOI | +60 | 3 × 10−11 |
Oxoacids
The acidity of oxoacids, with the general formula HOXOn (with n = 0−3), depends strongly on the number of terminal oxygen atoms (oxygen atoms only bonded to the central atom X).
Because oxygen is the second most electronegative element, adding terminal oxygen atoms causes strong inductive effect, thereby increasing the strength of the acid. For example, the figure below shows how the H atom in the acids becomes steadily more δ+ (more blue) from HClO to HClO4, making it easier for the acid to lose the hydrogen as an H+ ion.
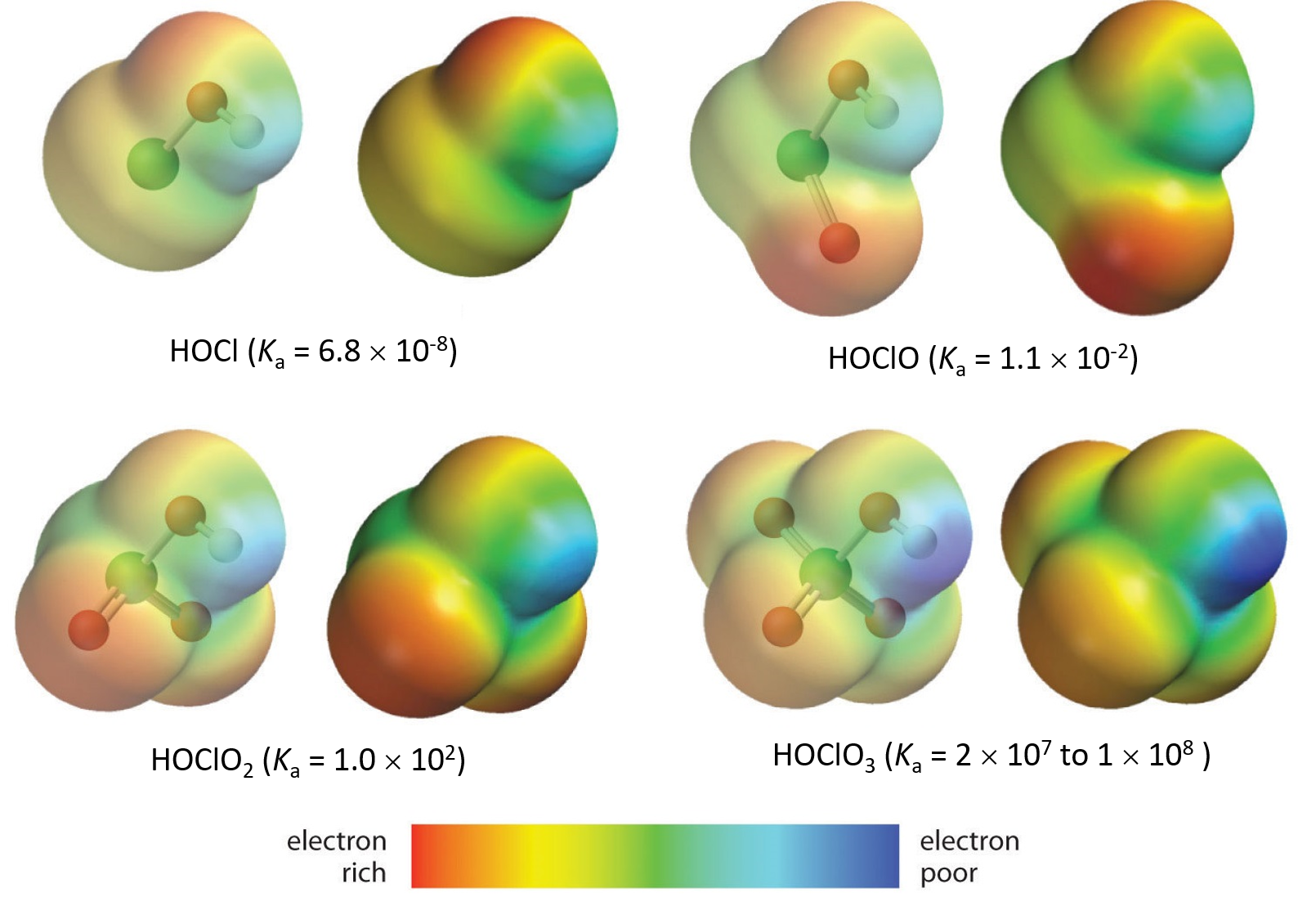
Also important is the effect of resonance stabilization in the conjugate base. For example, in the chlorite anion (ClO2–), the excess electron density is delocalized equally over both oxygen atoms, whereas in the hypochlorite ion (ClO–), the negative charge is largely localized on a single oxygen atom:
As a result of resonance stabilization and inductive effect, HClO2 is more than 200,000 times more acidic than HClO.
If we consider a series of oxoacids, we see that H3PO4 is a weak acid (Ka = 7.2 × 10−3), H2SO4 is a very strong acid (Ka = 4.0 × 103), and HClO4 (Ka = 3 × 107) is one of the strongest acids known. This trend is explained by the number of terminal oxygen atoms, which increases steadily across from P to Cl, consistent with the observed increase in acidity. In addition, the electronegativity of the central atom also increases, which further enhances the inductive effect on top of those from the terminal oxygen(s).
Please use this form to report any inconsistencies, errors, or other things you would like to change about this page. We appreciate your comments. 🙂