D41.4 Electrolysis
In an electrolytic cell, supplied electrical energy causes a nonspontaneous redox reaction to occur in a process known as electrolysis. An electrolytic cell is the opposite of a voltaic cell, where a spontaneous redox reaction produces electrical energy.
For example, consider electrolysis of molten sodium chloride. A simplified diagram of the electrolytic cell used for commercial manufacture of sodium metal and chlorine gas is shown below. In the molten salt, Na+ ions move toward the cathode and Cl– ions move toward the anode (note that negative ions move through the circuit in the same overall clockwise direction as electrons). A porous screen allows movement of ions but prevents mixing of the products (Na(s) and Cl2(g)), which would react spontaneously upon contact.
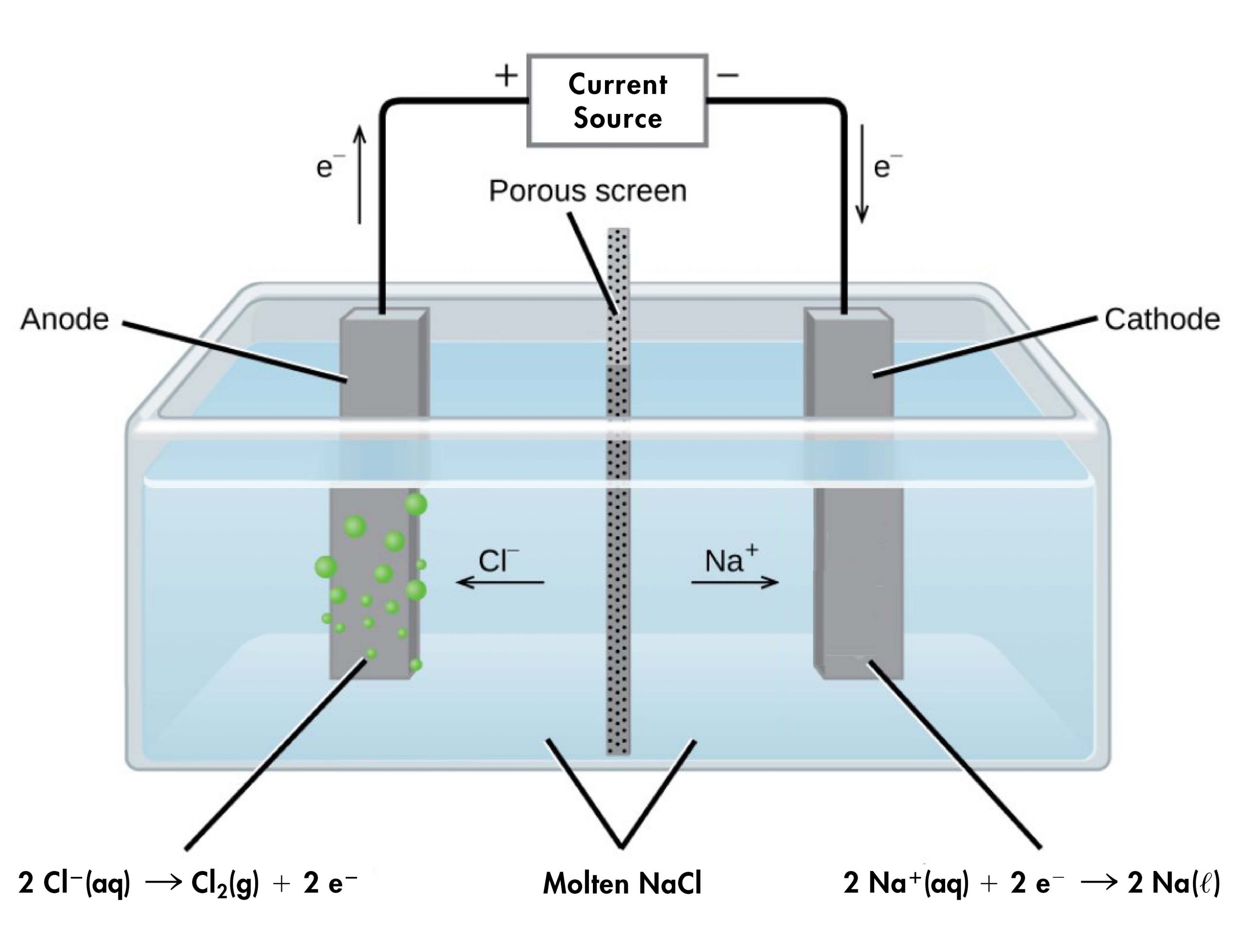
An external source of electric current forces electrons into the electrode in the cathode compartment, forcing the reduction half-reaction to occur:
(At the temperature of molten NaCl, sodium metal is a liquid, so E°Na+|Na(s) = -2.714 V is an approximation. E°Na+|Na(ℓ) for liquid sodium is not available in the appendix.)
In the anode compartment, the oxidation half-reaction occurs:
The electrons formed here are conducted through a wire to the the positive terminal of the voltage source, completing the electric circuit.
We can calculate E°cell for this electrolysis cell using the same method we used for voltaic cells:
The overall reaction is:
The negative E°cell indicates that this reaction is strongly reactant-favored, and under standard-state conditions, the power supply must provide at least 4.1 V to cause the electrolysis reaction to occur. In practice, the applied voltages are higher due to inefficiencies in the process itself and also to help increase the rate of the reaction.
With the transition from fossil fuels to renewable energy supplies, an important application of electrolysis is the “splitting” of water into hydrogen gas and oxygen gas. Electric energy from solar panels or wind turbines can be used to synthesize H2(g) for use as a fuel. For electric current to pass through the solution efficiently, there must be ions present. Hence, acid is typically added to the reaction solution to increase the concentrations of ions in solution.
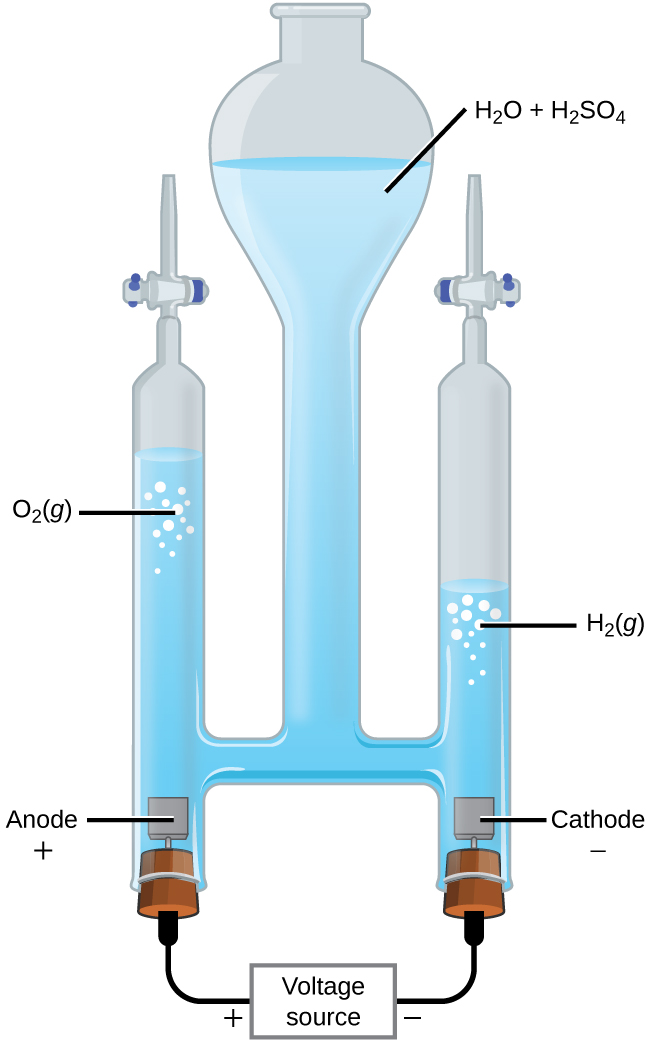
In a 1-M acidic solution:
Oxidation (anode): | 2 H2O(ℓ) | ⟶ | O2(g) + 4 H+(aq) + 4 e– | E°anode = +1.229 V |
Reduction (cathode): | 2 × [2 H+(aq) + 2 e– | ⟶ | H2(g)] | E°cathode = 0 V |
Overall: | 2 H2O(ℓ) | ⟶ | O2(g) + 2 H2(g) | E°cell = -1.229 V |
At least 1.229 V is required to make this reactant-favored process occur in a 1-M acidic solution.
In the last example, let’s consider what occurs during the electrolysis of 1-M aqueous potassium iodide solution at 25 °C. Present in the solution are H2O(ℓ), K+(aq), and I–(aq). This example differs from the previous two examples because more than one species can be oxidized and more than one species can be reduced.
Considering the anode first, the possible oxidation reactions are (oxidation of K+ to K2+ is not considered because K+ has a noble-gas electron configuration and a very high ionization energy, making it very difficult to oxidize):
(i) | 2 I–(aq) | ⟶ | I2(s) + 2 e– | E°anode = +0.535 V |
(ii) | 2 H2O(ℓ) | ⟶ | O2(g) + 4 H+(aq) + 4 e– | E°anode = +1.229 V |
(Note that although I2 is generated in aqueous solution, we approximate the E° here by using the value for I2(s).)
The pH of a 1-M KI solution is 7, so [H+] is far from standard-state conditions. Assuming that O2 is produced at 1 bar, applying the Nernst equation to half-reaction (ii) gives:
![Rendered by QuickLaTeX.com \begin{array}{rcl} E &=& {E^ \circ } - \dfrac{RT}{nF}\ln \left( \dfrac{1}{[\text{O}_2][\text{H}^+]^4}\right)\\[1.5em] &=& 1.229\text{ V} - \dfrac{\left(8.314 \frac{\text{J}}{\text{K}\cdot\text{mol}}\right) (298.15\;\text{K})}{4\left(96485\frac{\text{J}}{\text{V}\cdot\text{mol}}\right)} \ln \left(\dfrac{1}{(1)(1 \times 10^{-7})^4}\right)\\[1.5em] &=& 1.229\;{\text{ V}} - 0.414\;\text{V}\; =\; 0.815\;\text{V} \end{array}](https://wisc.pb.unizin.org/app/uploads/quicklatex/quicklatex.com-6a94ab3eabc095f37e203a4d7d894f45_l3.png)
(Note that the reaction given in the standard half-cell potential table is the reduction reaction “O2(g) + 4H+(aq) + 4e– ⟶ 2H2O(l) E° = +1.229 V”, hence reaction quotient Q is expressed in accordance to the given reaction.)
Because E°cell = E°cathode – E°anode, a more positive anode half-cell potential would lead to a more negative overall cell potential. The Nernst equation shows that Eanode = +0.815 V for reaction (ii) at pH = 7, which is higher (more positive) than E°anode for reaction (i). Therefore, iodide is the species being oxidized at the anode and I2 forms as a product.
Now consider the possible reactions at the cathode (reduction of I– is not considered because I– has a noble-gas electron configuration and it is not energetically favorable to add more electrons):
(iii) | 2 H2O(ℓ) + 2e– | ⟶ | H2(g) + 2 OH–(aq) | E°cathode = -0.8277 V |
(iv) | K+(aq) + e– | ⟶ | K(s) | E°cathode = -2.925 V |
For half-reaction (iii), we again need to apply the Nernst equation to calculate Ecathode at pH = 7, assuming H2 is produced at 1 bar:
![Rendered by QuickLaTeX.com \begin{array}{rcl} E &=& {E^ \circ } - \dfrac{RT}{nF}\ln \left( \dfrac{[\text{H}_2][\text{OH}^-]^2}{1}\right)\\[1.5em] &=& -0.8277\text{ V} - \dfrac{\left(8.314 \frac{\text{J}}{\text{K}\cdot\text{mol}}\right) (298.15\;\text{K})}{2\left(96485\frac{\text{J}}{\text{V}\cdot\text{mol}}\right)} \ln \left(\dfrac{(1)(1 \times 10^{-7})^2}{1}\right)\\[1.5em] &=& -0.8277\;{\text{ V}} - (-0.4141\;\text{V})\; =\; -0.4136\;\text{V} \end{array}](https://wisc.pb.unizin.org/app/uploads/quicklatex/quicklatex.com-d6fa987c2c8b0d04b78e5188331335e9_l3.png)
Hence, reduction of water, with Ecathode = -0.4136 V at pH = 7, is much more likely to occur than reduction of K+(aq) with E°cathode = -2.925 V. This conclusion is supported by the fact that potassium metal would react vigorously with water to form K+(aq), H2(g), and OH–(aq), so if K(s) were to form, it would immediately react with water.
Therefore, the overall reaction occurring in this electrolytic cell is:
Oxidation (anode): | 2 I–(aq) | ⟶ | I2(s) + 2e– | E°anode = +0.535 V |
Reduction (cathode): | 2 H2O(ℓ) + 2 e– | ⟶ | H2(g) + 2 OH–(aq) | Ecathode = -0.4136 V |
Overall: | 2 H2O(ℓ) + 2 I–(aq) | ⟶ | H2(g) + I2(s) + 2 OH–(aq) | Ecell = -0.949 V |
Please use this form to report any inconsistencies, errors, or other things you would like to change about this page. We appreciate your comments. 🙂